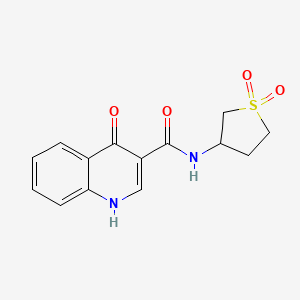
N-(1,1-dioxidotetrahydro-3-thienyl)-4-hydroxy-3-quinolinecarboxamide
- Click on QUICK INQUIRY to receive a quote from our team of experts.
- With the quality product at a COMPETITIVE price, you can focus more on your research.
Overview
Description
N-(1,1-dioxidotetrahydro-3-thienyl)-4-hydroxy-3-quinolinecarboxamide is a chemical compound that has been widely studied for its potential applications in scientific research. This compound is known for its unique structure and properties, which make it a valuable tool for investigating various biological processes.
Scientific Research Applications
G Protein-Gated Inwardly-Rectifying Potassium (GIRK) Channel Activation
- Application : Researchers have discovered that N-(1,1-dioxidotetrahydrothiophen-3-yl)-4-hydroxyquinoline-3-carboxamide ethers act as novel GIRK1/2 channel activators. These compounds enhance potassium ion flow through GIRK channels, potentially influencing neuronal excitability, pain perception, and other physiological processes .
Improved Metabolic Stability Over Urea-Based Compounds
- Application : N-(1,1-dioxidotetrahydrothiophen-3-yl)-4-hydroxyquinoline-3-carboxamide ethers have demonstrated nanomolar potency as GIRK1/2 activators while showing improved metabolic stability compared to prototypical urea-based compounds. This finding suggests their potential as pharmacological tools for studying GIRK channels and therapeutic targets .
Potential Therapeutic Indications
- Application : While more research is needed, GIRK channels could be potential targets for indications such as pain perception, epilepsy, reward/addiction, and anxiety. The selective and brain-penetrant properties of these compounds make them valuable tools for further investigation .
Chemical Structure and Synonyms
- Synonyms : Apart from the full name, it is also known as N-(1,1-dioxidotetrahydro-3-thienyl)-4-hydroxy-3-quinolinecarboxamide. Its CAS number is 462069-23-4.
Mechanism of Action
Target of Action
The primary target of N-(1,1-dioxidotetrahydrothiophen-3-yl)-4-hydroxyquinoline-3-carboxamide is the G protein-gated inwardly-rectifying potassium (GIRK) channels . These channels are key effectors in GPCR signaling pathways that modulate excitability in cells .
Mode of Action
This compound acts as an activator of the GIRK channels . It interacts with these channels, leading to their activation and subsequent changes in cell excitability .
Biochemical Pathways
The activation of GIRK channels affects various biochemical pathways. GIRK channels are involved in numerous physiological processes and potential targets for various indications, such as pain perception, epilepsy, reward/addiction, and anxiety .
Result of Action
The activation of GIRK channels by this compound leads to changes in cell excitability. This can have various effects at the molecular and cellular level, depending on the specific physiological context .
properties
IUPAC Name |
N-(1,1-dioxothiolan-3-yl)-4-oxo-1H-quinoline-3-carboxamide |
Source
|
---|---|---|
Details | Computed by Lexichem TK 2.7.0 (PubChem release 2021.05.07) | |
Source | PubChem | |
URL | https://pubchem.ncbi.nlm.nih.gov | |
Description | Data deposited in or computed by PubChem | |
InChI |
InChI=1S/C14H14N2O4S/c17-13-10-3-1-2-4-12(10)15-7-11(13)14(18)16-9-5-6-21(19,20)8-9/h1-4,7,9H,5-6,8H2,(H,15,17)(H,16,18) |
Source
|
Details | Computed by InChI 1.0.6 (PubChem release 2021.05.07) | |
Source | PubChem | |
URL | https://pubchem.ncbi.nlm.nih.gov | |
Description | Data deposited in or computed by PubChem | |
InChI Key |
KTGUQIPJSTUZDS-UHFFFAOYSA-N |
Source
|
Details | Computed by InChI 1.0.6 (PubChem release 2021.05.07) | |
Source | PubChem | |
URL | https://pubchem.ncbi.nlm.nih.gov | |
Description | Data deposited in or computed by PubChem | |
Canonical SMILES |
C1CS(=O)(=O)CC1NC(=O)C2=CNC3=CC=CC=C3C2=O |
Source
|
Details | Computed by OEChem 2.3.0 (PubChem release 2021.05.07) | |
Source | PubChem | |
URL | https://pubchem.ncbi.nlm.nih.gov | |
Description | Data deposited in or computed by PubChem | |
Molecular Formula |
C14H14N2O4S |
Source
|
Details | Computed by PubChem 2.1 (PubChem release 2021.05.07) | |
Source | PubChem | |
URL | https://pubchem.ncbi.nlm.nih.gov | |
Description | Data deposited in or computed by PubChem | |
Molecular Weight |
306.34 g/mol |
Source
|
Details | Computed by PubChem 2.1 (PubChem release 2021.05.07) | |
Source | PubChem | |
URL | https://pubchem.ncbi.nlm.nih.gov | |
Description | Data deposited in or computed by PubChem | |
Product Name |
N-(1,1-dioxidotetrahydrothiophen-3-yl)-4-hydroxyquinoline-3-carboxamide |
Disclaimer and Information on In-Vitro Research Products
Please be aware that all articles and product information presented on BenchChem are intended solely for informational purposes. The products available for purchase on BenchChem are specifically designed for in-vitro studies, which are conducted outside of living organisms. In-vitro studies, derived from the Latin term "in glass," involve experiments performed in controlled laboratory settings using cells or tissues. It is important to note that these products are not categorized as medicines or drugs, and they have not received approval from the FDA for the prevention, treatment, or cure of any medical condition, ailment, or disease. We must emphasize that any form of bodily introduction of these products into humans or animals is strictly prohibited by law. It is essential to adhere to these guidelines to ensure compliance with legal and ethical standards in research and experimentation.