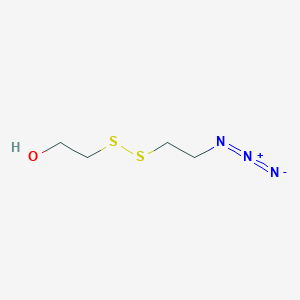
Azidoethyl-SS-ethylalcohol
Overview
Description
It is a bifunctional linker molecule that consists of an azido functional group, a disulfide linker, and an ethyl alcohol functional group. The azido group enables Click Chemistry, while the hydroxyl group allows further derivatization or replacement with other reactive functional groups.
Mechanism of Action
Target of Action
Azidoethyl-SS-ethylalcohol is a Click Chemistry crosslinker . It contains a hydroxyl group, a cleavable disulfide bond, and an azide group . The primary targets of this compound are proteins, peptides, and small molecules . The compound is used to modify these targets for various applications, such as drug delivery, imaging, and diagnostics .
Mode of Action
The azide group in this compound enables Click Chemistry . Click Chemistry is a type of chemical reaction that joins small units together in a wide variety of materials under mild conditions. The hydroxyl group in the compound allows for further derivatization or replacement with other reactive functional groups . This means that the compound can interact with its targets and cause changes in their structure or function.
Biochemical Pathways
Given its role as a crosslinker in click chemistry, it can be inferred that the compound may be involved in the modification of proteins, peptides, and small molecules . These modifications can potentially affect various biochemical pathways, depending on the specific targets and the nature of the modifications.
Result of Action
The molecular and cellular effects of this compound’s action would depend on the specific targets and the modifications made to them. As a crosslinker, the compound could potentially alter the structure and function of its targets, leading to various molecular and cellular effects .
Action Environment
Environmental factors can influence the action, efficacy, and stability of this compound. For instance, the compound is recommended to be stored at -20°C , suggesting that temperature could affect its stability. Other environmental factors, such as pH and the presence of other chemicals, could also potentially influence the compound’s action and efficacy.
Biochemical Analysis
Biochemical Properties
Azidoethyl-SS-ethylalcohol plays a significant role in biochemical reactions due to its unique structure. The azide group enables Click Chemistry, a powerful tool for bioconjugation, allowing for the creation of complex molecules through simple and efficient reactions . The hydroxyl group provides versatility, enabling further derivatization or replacement with other reactive functional groups
Cellular Effects
It is known that the compound’s azide group enables Click Chemistry, which can be used to modify cellular components, potentially influencing cell function
Molecular Mechanism
The molecular mechanism of this compound is primarily based on its role as a Click Chemistry crosslinker . The azide group in the compound can react with alkynes or cyclooctynes in a [3+2] cycloaddition to form a stable triazole ring, a reaction that is often used in bioconjugation . This allows for the creation of complex molecules through simple and efficient reactions
Preparation Methods
Synthetic Routes and Reaction Conditions
Azidoethyl-SS-ethylalcohol is synthesized through a series of chemical reactions involving the introduction of azido and disulfide groups to an ethyl alcohol backbone. The synthesis typically involves the following steps:
Formation of the Disulfide Linker: The initial step involves the formation of a disulfide bond between two thiol groups. This can be achieved through oxidation reactions using reagents such as hydrogen peroxide or iodine.
Introduction of the Azido Group: The azido group is introduced through nucleophilic substitution reactions. Sodium azide is commonly used as the azide source, reacting with an appropriate leaving group on the ethyl alcohol derivative.
Final Product Formation: The final product, this compound, is obtained by combining the disulfide linker with the azido-functionalized ethyl alcohol.
Industrial Production Methods
Industrial production of this compound follows similar synthetic routes but on a larger scale. The process involves optimizing reaction conditions to ensure high yield and purity. Key considerations include:
Reaction Temperature and Time: Controlling the temperature and reaction time to maximize product formation while minimizing side reactions.
Purification: Employing techniques such as chromatography and recrystallization to purify the final product.
Safety Measures: Implementing safety protocols to handle hazardous reagents like sodium azide and to manage the exothermic nature of the reactions.
Chemical Reactions Analysis
Types of Reactions
Azidoethyl-SS-ethylalcohol undergoes various chemical reactions, including:
Click Chemistry: The azido group reacts with alkyne-functionalized molecules to form stable triazole linkages through a copper-catalyzed azide-alkyne cycloaddition reaction.
Reduction: The disulfide bond can be cleaved under reducing conditions, releasing the target molecule.
Substitution: The hydroxyl group can be replaced with other reactive functional groups for further derivatization.
Common Reagents and Conditions
Copper-Catalyzed Azide-Alkyne Cycloaddition: Copper sulfate and sodium ascorbate are commonly used as catalysts for Click Chemistry reactions.
Reducing Agents: Dithiothreitol (DTT) and tris(2-carboxyethyl)phosphine (TCEP) are used to cleave the disulfide bond.
Nucleophilic Substitution: Sodium azide is used for introducing the azido group.
Major Products
Triazole Linkages: Formed through Click Chemistry reactions.
Cleaved Disulfide Products: Resulting from reduction reactions.
Scientific Research Applications
Azidoethyl-SS-ethylalcohol has a wide range of applications in scientific research, including:
Chemistry: Used as a crosslinker in Click Chemistry for the synthesis of complex molecules.
Biology: Employed in bioconjugation to label biomolecules such as proteins and nucleic acids.
Medicine: Utilized in drug delivery systems to attach therapeutic agents to targeting molecules.
Industry: Applied in the development of diagnostic tools and imaging agents.
Comparison with Similar Compounds
Azidoethyl-SS-ethylalcohol is unique due to its combination of azido, disulfide, and ethyl alcohol functional groups. Similar compounds include:
Azidoethyl-SS-ethylamine: Contains an amine group instead of an alcohol group.
Azidoethyl-SS-ethylcarboxylate: Contains a carboxylate group instead of an alcohol group.
Azidoethyl-SS-ethylthiol: Contains a thiol group instead of an alcohol group.
These similar compounds share the azido and disulfide functionalities but differ in their additional functional groups, which can influence their reactivity and applications.
Properties
IUPAC Name |
2-(2-azidoethyldisulfanyl)ethanol | |
---|---|---|
Source | PubChem | |
URL | https://pubchem.ncbi.nlm.nih.gov | |
Description | Data deposited in or computed by PubChem | |
InChI |
InChI=1S/C4H9N3OS2/c5-7-6-1-3-9-10-4-2-8/h8H,1-4H2 | |
Source | PubChem | |
URL | https://pubchem.ncbi.nlm.nih.gov | |
Description | Data deposited in or computed by PubChem | |
InChI Key |
NVUXJTRJLAYMKH-UHFFFAOYSA-N | |
Source | PubChem | |
URL | https://pubchem.ncbi.nlm.nih.gov | |
Description | Data deposited in or computed by PubChem | |
Canonical SMILES |
C(CSSCCO)N=[N+]=[N-] | |
Source | PubChem | |
URL | https://pubchem.ncbi.nlm.nih.gov | |
Description | Data deposited in or computed by PubChem | |
Molecular Formula |
C4H9N3OS2 | |
Source | PubChem | |
URL | https://pubchem.ncbi.nlm.nih.gov | |
Description | Data deposited in or computed by PubChem | |
Molecular Weight |
179.3 g/mol | |
Source | PubChem | |
URL | https://pubchem.ncbi.nlm.nih.gov | |
Description | Data deposited in or computed by PubChem | |
Retrosynthesis Analysis
AI-Powered Synthesis Planning: Our tool employs the Template_relevance Pistachio, Template_relevance Bkms_metabolic, Template_relevance Pistachio_ringbreaker, Template_relevance Reaxys, Template_relevance Reaxys_biocatalysis model, leveraging a vast database of chemical reactions to predict feasible synthetic routes.
One-Step Synthesis Focus: Specifically designed for one-step synthesis, it provides concise and direct routes for your target compounds, streamlining the synthesis process.
Accurate Predictions: Utilizing the extensive PISTACHIO, BKMS_METABOLIC, PISTACHIO_RINGBREAKER, REAXYS, REAXYS_BIOCATALYSIS database, our tool offers high-accuracy predictions, reflecting the latest in chemical research and data.
Strategy Settings
Precursor scoring | Relevance Heuristic |
---|---|
Min. plausibility | 0.01 |
Model | Template_relevance |
Template Set | Pistachio/Bkms_metabolic/Pistachio_ringbreaker/Reaxys/Reaxys_biocatalysis |
Top-N result to add to graph | 6 |
Feasible Synthetic Routes
Disclaimer and Information on In-Vitro Research Products
Please be aware that all articles and product information presented on BenchChem are intended solely for informational purposes. The products available for purchase on BenchChem are specifically designed for in-vitro studies, which are conducted outside of living organisms. In-vitro studies, derived from the Latin term "in glass," involve experiments performed in controlled laboratory settings using cells or tissues. It is important to note that these products are not categorized as medicines or drugs, and they have not received approval from the FDA for the prevention, treatment, or cure of any medical condition, ailment, or disease. We must emphasize that any form of bodily introduction of these products into humans or animals is strictly prohibited by law. It is essential to adhere to these guidelines to ensure compliance with legal and ethical standards in research and experimentation.