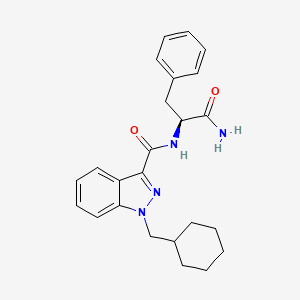
App-chminaca
Overview
Description
Mechanism of Action
Target of Action
APP-CHMINACA, also known as PX-3, is an indazole-based synthetic cannabinoid . It primarily targets the CB1 receptor , a type of cannabinoid receptor in the human body . The CB1 receptor plays a crucial role in the regulation of neurotransmission in the brain, and its activation can lead to various psychoactive effects.
Mode of Action
This compound acts as a potent agonist for the CB1 receptor . An agonist is a substance that initiates a physiological response when combined with a receptor. In this case, this compound binds to the CB1 receptor with a high affinity, triggering a response that can include pain relief, relaxation, and altered perception.
Biochemical Pathways
The primary biochemical transformations observed for this compound are the hydrolysis of the distal amide group and the hydroxylation of the cyclohexylmethyl (CHM) substituent . These transformations lead to the production of various metabolites, some of which are specific to this compound . The metabolites in greatest abundance in the study were products of amide hydrolysis with no further biotransformation (M1), followed by amide hydrolysis with monohydroxylation (M2.1) .
Result of Action
The activation of the CB1 receptor by this compound leads to various molecular and cellular effects. These can include alterations in neurotransmission, changes in perception, and potential analgesic effects . .
Action Environment
The action, efficacy, and stability of this compound can be influenced by various environmental factors. While specific studies on this compound are limited, research on similar synthetic cannabinoids suggests that factors such as temperature, humidity, and light intensity can affect the stability and potency of these compounds . Furthermore, the body’s internal environment, including factors like pH levels and the presence of other substances, can also impact the action of this compound.
Biochemical Analysis
Biochemical Properties
APP-CHMINACA interacts with cannabinoid receptors, specifically cannabinoid receptor 1 (CB1) and cannabinoid receptor 2 (CB2) . It has a high affinity to bind to these receptors and is estimated to be more potent than tetrahydrocannabinol (THC) in mice .
Cellular Effects
This compound can have significant effects on various types of cells and cellular processes. For instance, it has been observed to cause congestion, hemorrhage, degeneration, and cellular infiltration of the liver and kidney tissues . It also influences cell function, including any impact on cell signaling pathways, gene expression, and cellular metabolism .
Molecular Mechanism
At the molecular level, this compound exerts its effects through binding interactions with biomolecules, enzyme inhibition or activation, and changes in gene expression . It primarily exerts its effects through activating the CB1 and CB2 receptors .
Temporal Effects in Laboratory Settings
In laboratory settings, the effects of this compound change over time. Chronic administration of this compound causes evident liver and kidney histotoxic effects even at low doses with unnoticeable clinical effects in mice .
Dosage Effects in Animal Models
The effects of this compound vary with different dosages in animal models. For instance, daily intraperitoneal injections of this compound at doses of 0.3 mg/kg, 3 mg/kg, or 10 mg/kg in adult male albino mice showed significant toxic effects on the liver and kidneys .
Metabolic Pathways
This compound is involved in various metabolic pathways. Incubation of this compound with human liver microsomes yielded 12 metabolites, encompassing 7 different metabolite classes .
Transport and Distribution
Its metabolites have been detected in the blood, indicating that it can be transported and distributed within the body .
Subcellular Localization
Given its interaction with cannabinoid receptors, it is likely that it localizes to the cell membrane where these receptors are typically found .
Preparation Methods
Synthetic Routes and Reaction Conditions
The synthesis of APP-CHMINACA involves several key steps. The starting material is typically an indazole-3-carboxylate ester, which undergoes alkylation to produce 1-alkyl-1H-indazole and 2-alkyl-2H-indazole intermediates . The regioselectivity of this reaction is primarily determined by the choice of base. The final product is obtained through a series of reactions including amide formation and cyclohexylmethylation .
Industrial Production Methods
Industrial production of this compound follows similar synthetic routes but on a larger scale. The process involves optimizing reaction conditions such as temperature, pressure, and solvent choice to maximize yield and purity. The use of automated reactors and continuous flow systems can enhance the efficiency and scalability of the production process .
Chemical Reactions Analysis
Types of Reactions
APP-CHMINACA undergoes various chemical reactions, including:
Oxidation: Hydroxylation of the cyclohexylmethyl group.
Reduction: Reduction of the carbonyl group to form alcohol derivatives.
Substitution: Nucleophilic substitution reactions at the indazole ring.
Common Reagents and Conditions
Oxidation: Common reagents include hydrogen peroxide and potassium permanganate.
Reduction: Sodium borohydride and lithium aluminum hydride are frequently used.
Substitution: Halogenated solvents and strong nucleophiles such as sodium hydride.
Major Products
The major products formed from these reactions include hydroxylated derivatives, reduced alcohols, and substituted indazole compounds .
Scientific Research Applications
APP-CHMINACA has several scientific research applications:
Comparison with Similar Compounds
Similar Compounds
PX-1 (5F-APP-PICA): Similar structure but with a fluoropentyl side chain.
PX-2 (5F-APP-PINACA): Contains an indazole core and a fluoropentyl side chain.
MDMB-CHMINACA: Another indazole-based synthetic cannabinoid with different substituents.
Uniqueness
APP-CHMINACA is unique due to its specific combination of an indazole core, a cyclohexylmethyl group, and an amide linkage. This structure confers high potency and selectivity for CB1 receptors, distinguishing it from other synthetic cannabinoids .
Properties
IUPAC Name |
N-[(2S)-1-amino-1-oxo-3-phenylpropan-2-yl]-1-(cyclohexylmethyl)indazole-3-carboxamide | |
---|---|---|
Source | PubChem | |
URL | https://pubchem.ncbi.nlm.nih.gov | |
Description | Data deposited in or computed by PubChem | |
InChI |
InChI=1S/C24H28N4O2/c25-23(29)20(15-17-9-3-1-4-10-17)26-24(30)22-19-13-7-8-14-21(19)28(27-22)16-18-11-5-2-6-12-18/h1,3-4,7-10,13-14,18,20H,2,5-6,11-12,15-16H2,(H2,25,29)(H,26,30)/t20-/m0/s1 | |
Source | PubChem | |
URL | https://pubchem.ncbi.nlm.nih.gov | |
Description | Data deposited in or computed by PubChem | |
InChI Key |
DMHWDSGURMXMGE-FQEVSTJZSA-N | |
Source | PubChem | |
URL | https://pubchem.ncbi.nlm.nih.gov | |
Description | Data deposited in or computed by PubChem | |
Canonical SMILES |
C1CCC(CC1)CN2C3=CC=CC=C3C(=N2)C(=O)NC(CC4=CC=CC=C4)C(=O)N | |
Source | PubChem | |
URL | https://pubchem.ncbi.nlm.nih.gov | |
Description | Data deposited in or computed by PubChem | |
Isomeric SMILES |
C1CCC(CC1)CN2C3=CC=CC=C3C(=N2)C(=O)N[C@@H](CC4=CC=CC=C4)C(=O)N | |
Source | PubChem | |
URL | https://pubchem.ncbi.nlm.nih.gov | |
Description | Data deposited in or computed by PubChem | |
Molecular Formula |
C24H28N4O2 | |
Source | PubChem | |
URL | https://pubchem.ncbi.nlm.nih.gov | |
Description | Data deposited in or computed by PubChem | |
DSSTOX Substance ID |
DTXSID201009998 | |
Record name | APP-CHMINACA | |
Source | EPA DSSTox | |
URL | https://comptox.epa.gov/dashboard/DTXSID201009998 | |
Description | DSSTox provides a high quality public chemistry resource for supporting improved predictive toxicology. | |
Molecular Weight |
404.5 g/mol | |
Source | PubChem | |
URL | https://pubchem.ncbi.nlm.nih.gov | |
Description | Data deposited in or computed by PubChem | |
CAS No. |
1185887-14-2 | |
Record name | N-[(1S)-2-Amino-2-oxo-1-(phenylmethyl)ethyl]-1-(cyclohexylmethyl)-1H-indazole-3-carboxamide | |
Source | CAS Common Chemistry | |
URL | https://commonchemistry.cas.org/detail?cas_rn=1185887-14-2 | |
Description | CAS Common Chemistry is an open community resource for accessing chemical information. Nearly 500,000 chemical substances from CAS REGISTRY cover areas of community interest, including common and frequently regulated chemicals, and those relevant to high school and undergraduate chemistry classes. This chemical information, curated by our expert scientists, is provided in alignment with our mission as a division of the American Chemical Society. | |
Explanation | The data from CAS Common Chemistry is provided under a CC-BY-NC 4.0 license, unless otherwise stated. | |
Record name | APP-CHMINACA | |
Source | ChemIDplus | |
URL | https://pubchem.ncbi.nlm.nih.gov/substance/?source=chemidplus&sourceid=1185887142 | |
Description | ChemIDplus is a free, web search system that provides access to the structure and nomenclature authority files used for the identification of chemical substances cited in National Library of Medicine (NLM) databases, including the TOXNET system. | |
Record name | APP-CHMINACA | |
Source | EPA DSSTox | |
URL | https://comptox.epa.gov/dashboard/DTXSID201009998 | |
Description | DSSTox provides a high quality public chemistry resource for supporting improved predictive toxicology. | |
Record name | APP-CHMINACA | |
Source | FDA Global Substance Registration System (GSRS) | |
URL | https://gsrs.ncats.nih.gov/ginas/app/beta/substances/3877T06H05 | |
Description | The FDA Global Substance Registration System (GSRS) enables the efficient and accurate exchange of information on what substances are in regulated products. Instead of relying on names, which vary across regulatory domains, countries, and regions, the GSRS knowledge base makes it possible for substances to be defined by standardized, scientific descriptions. | |
Explanation | Unless otherwise noted, the contents of the FDA website (www.fda.gov), both text and graphics, are not copyrighted. They are in the public domain and may be republished, reprinted and otherwise used freely by anyone without the need to obtain permission from FDA. Credit to the U.S. Food and Drug Administration as the source is appreciated but not required. | |
Q1: What are the major metabolic pathways of APP-CHMINACA in humans?
A1: Research using human liver microsomes revealed that this compound undergoes extensive phase I metabolism, primarily through amide hydrolysis and hydroxylation []. The most common biotransformations were hydrolysis of the amide group and hydroxylation of the cyclohexylmethyl substituent. Notably, three specific metabolites were identified as unique markers for this compound consumption: a mono-, di-, and tri-hydroxylated metabolite on the cyclohexylmethyl group [].
Q2: What are the key analytical methods used to identify and quantify this compound and its metabolites?
A2: The research utilized high-resolution mass spectrometry (HRMS) to analyze extracts from in vitro studies with human liver microsomes []. This technique allowed for the identification and characterization of this compound metabolites based on their product ion spectra, accurate mass, and chemical formula. These methods are crucial for analytical scientists developing new methods for detecting this compound in biological samples.
Disclaimer and Information on In-Vitro Research Products
Please be aware that all articles and product information presented on BenchChem are intended solely for informational purposes. The products available for purchase on BenchChem are specifically designed for in-vitro studies, which are conducted outside of living organisms. In-vitro studies, derived from the Latin term "in glass," involve experiments performed in controlled laboratory settings using cells or tissues. It is important to note that these products are not categorized as medicines or drugs, and they have not received approval from the FDA for the prevention, treatment, or cure of any medical condition, ailment, or disease. We must emphasize that any form of bodily introduction of these products into humans or animals is strictly prohibited by law. It is essential to adhere to these guidelines to ensure compliance with legal and ethical standards in research and experimentation.