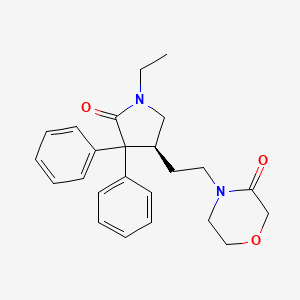
Ketodoxapram, (S)-
Overview
Description
Ketodoxapram, (S)- is a complex organic compound with significant applications in various fields, including medicinal chemistry and industrial processes. This compound is characterized by its unique structure, which includes a morpholinone ring and a pyrrolidinyl group, making it a subject of interest for researchers and chemists.
Scientific Research Applications
Ketodoxapram, (S)- has a wide range of applications in scientific research:
Chemistry: Used as an intermediate in the synthesis of complex organic molecules.
Biology: Investigated for its potential as an antimicrobial and antifungal agent.
Medicine: Explored for its role in drug development, particularly in the synthesis of urea derivatives with potential therapeutic properties.
Industry: Utilized in the production of various chemicals and materials due to its versatile reactivity.
Mechanism of Action
Target of Action
Ketodoxapram, also known as “VL0W61GNK6” or “AHR-5955, (-)-”, primarily targets the TASK-1 channel . TASK-1 is a potassium channel that is almost exclusively expressed in the atria of the heart . It plays a significant role in the shortening of the atrial action potential observed during atrial fibrillation (AF), making it a promising target for AF therapy .
Mode of Action
Ketodoxapram interacts with its target, the TASK-1 channel, by inhibiting it . This inhibition is thought to stimulate the peripheral carotid chemoreceptors . The compound and its parent drug, doxapram, show strong inhibitory effects on TASK-1 .
Biochemical Pathways
The inhibition of the TASK-1 channel by ketodoxapram affects the biochemical pathways involved in atrial fibrillation. TASK-1 is significantly upregulated in AF patients . By inhibiting this channel, ketodoxapram can potentially terminate AF and restore sinus rhythm .
Pharmacokinetics
Ketodoxapram exhibits a longer terminal half-life (t1/2) of 1.71 hours compared to doxapram’s 1.38 hours . It also has a higher maximal concentration (Cmax; 4,604 ng/ml) than doxapram (1,780 ng/ml) . The brain-to-plasma ratio for ketodoxapram (0.065) hints at a reduced crossing of the blood-brain barrier (BBB) for ketodoxapram compared to doxapram (0.58) . These properties suggest that ketodoxapram may have superior bioavailability and efficacy in the treatment of AF compared to doxapram .
Result of Action
The molecular and cellular effects of ketodoxapram’s action include a significant reduction in AF burdens . This is achieved through the inhibition of the TASK-1 channel, which leads to the termination of AF and the restoration of sinus rhythm .
Action Environment
The action, efficacy, and stability of ketodoxapram can be influenced by various environmental factors. For instance, the compound’s ability to cross the BBB is an important factor that can affect its action . Furthermore, the compound’s pharmacokinetics, such as its half-life and maximal concentration, can also be influenced by factors such as the dosage administered and the individual’s metabolic rate .
Biochemical Analysis
Biochemical Properties
Ketodoxapram, (S)-, and its parent compound doxapram have been shown to have strong inhibitory effects on TASK-1 and TASK-3 channels . These channels are almost exclusively expressed in the atria of the heart and play a crucial role in the shortening of the atrial action potential observed during AF . The inhibition of these channels by Ketodoxapram, (S)-, suggests its potential role in biochemical reactions related to AF therapy .
Cellular Effects
In the context of AF, Ketodoxapram, (S)-, has been shown to reduce AF burdens in a porcine model, comparable to doxapram . This suggests that Ketodoxapram, (S)-, may influence cell function by modulating ion channel activity, which could impact cell signaling pathways and cellular metabolism .
Molecular Mechanism
The molecular mechanism of action of Ketodoxapram, (S)-, is related to its inhibitory effects on TASK-1 and TASK-3 channels . These channels are involved in the regulation of the atrial action potential, and their inhibition by Ketodoxapram, (S)-, could lead to changes in gene expression and enzyme activity related to AF .
Temporal Effects in Laboratory Settings
It has been observed that Ketodoxapram, (S)-, has a longer terminal half-life and higher maximal concentration compared to doxapram in a porcine model . This suggests that the effects of Ketodoxapram, (S)-, may persist for a longer period compared to doxapram .
Dosage Effects in Animal Models
In animal models, the effects of Ketodoxapram, (S)-, have been studied at a dosage of 1 mg/kg . At this dosage, Ketodoxapram, (S)-, led to a significant reduction in AF burdens in a porcine model, comparable to doxapram
Metabolic Pathways
Ketodoxapram, (S)-, is a metabolite of doxapram, produced through the process of hydroxylation
Transport and Distribution
It has been observed that the brain-to-plasma ratio for Ketodoxapram, (S)-, is lower than for doxapram, suggesting a reduced crossing of the blood-brain barrier for Ketodoxapram, (S)- .
Subcellular Localization
Given its role as an inhibitor of TASK-1 and TASK-3 channels, it is likely that Ketodoxapram, (S)-, interacts with these channels at the cell membrane
Preparation Methods
Synthetic Routes and Reaction Conditions
The synthesis of Ketodoxapram, (S)- typically involves multiple steps. One common method includes the reaction of 2-(2-chloroethoxy)ethanol with 4-nitroaniline in the presence of a phenylboronic acid catalyst. This reaction produces 2-(2-chloroethoxy)-N-(4-nitrophenyl)acetamide, which is then transformed into 4-(4-nitrophenyl)morpholin-3-one through a one-pot procedure. The final step involves hydrogenation to yield 4-(4-aminophenyl)morpholin-3-one .
Industrial Production Methods
Industrial production of this compound often employs continuous-flow synthesis techniques to enhance efficiency and yield. These methods involve the use of specific catalysts and reaction conditions to ensure the purity and consistency of the final product .
Chemical Reactions Analysis
Types of Reactions
Ketodoxapram, (S)- undergoes various chemical reactions, including:
Oxidation: This reaction typically involves the use of oxidizing agents such as sodium hypochlorite.
Reduction: Commonly performed using hydrogenation with palladium on carbon (Pd-C) as a catalyst.
Substitution: Involves the replacement of functional groups under specific conditions.
Common Reagents and Conditions
Oxidizing Agents: Sodium hypochlorite, calcium hypochlorite.
Reducing Agents: Hydrogen gas with Pd-C catalyst.
Substitution Reagents: Phenylboronic acid, various amines.
Major Products
The major products formed from these reactions include various derivatives of morpholinone, which are used as intermediates in the synthesis of pharmaceuticals and other organic compounds .
Comparison with Similar Compounds
Similar Compounds
4-(4-Aminophenyl)morpholin-3-one: A key intermediate in the synthesis of rivaroxaban, an anticoagulant drug.
Metolachlor morpholinone: Used as a herbicide and has a similar morpholinone structure.
3-Morpholinone: A simpler analog with applications in organic synthesis.
Uniqueness
Ketodoxapram, (S)- stands out due to its complex structure, which imparts unique reactivity and biological activity. Its ability to form derivatives with diverse pharmacological properties makes it a valuable compound in medicinal chemistry and drug development.
Properties
IUPAC Name |
4-[2-[(3S)-1-ethyl-5-oxo-4,4-diphenylpyrrolidin-3-yl]ethyl]morpholin-3-one | |
---|---|---|
Source | PubChem | |
URL | https://pubchem.ncbi.nlm.nih.gov | |
Description | Data deposited in or computed by PubChem | |
InChI |
InChI=1S/C24H28N2O3/c1-2-25-17-21(13-14-26-15-16-29-18-22(26)27)24(23(25)28,19-9-5-3-6-10-19)20-11-7-4-8-12-20/h3-12,21H,2,13-18H2,1H3/t21-/m1/s1 | |
Source | PubChem | |
URL | https://pubchem.ncbi.nlm.nih.gov | |
Description | Data deposited in or computed by PubChem | |
InChI Key |
LLCHHQQQMSDZLP-OAQYLSRUSA-N | |
Source | PubChem | |
URL | https://pubchem.ncbi.nlm.nih.gov | |
Description | Data deposited in or computed by PubChem | |
Canonical SMILES |
CCN1CC(C(C1=O)(C2=CC=CC=C2)C3=CC=CC=C3)CCN4CCOCC4=O | |
Source | PubChem | |
URL | https://pubchem.ncbi.nlm.nih.gov | |
Description | Data deposited in or computed by PubChem | |
Isomeric SMILES |
CCN1C[C@H](C(C1=O)(C2=CC=CC=C2)C3=CC=CC=C3)CCN4CCOCC4=O | |
Source | PubChem | |
URL | https://pubchem.ncbi.nlm.nih.gov | |
Description | Data deposited in or computed by PubChem | |
Molecular Formula |
C24H28N2O3 | |
Source | PubChem | |
URL | https://pubchem.ncbi.nlm.nih.gov | |
Description | Data deposited in or computed by PubChem | |
Molecular Weight |
392.5 g/mol | |
Source | PubChem | |
URL | https://pubchem.ncbi.nlm.nih.gov | |
Description | Data deposited in or computed by PubChem | |
CAS No. |
1415394-64-7 | |
Record name | AHR-5955, (S)- | |
Source | ChemIDplus | |
URL | https://pubchem.ncbi.nlm.nih.gov/substance/?source=chemidplus&sourceid=1415394647 | |
Description | ChemIDplus is a free, web search system that provides access to the structure and nomenclature authority files used for the identification of chemical substances cited in National Library of Medicine (NLM) databases, including the TOXNET system. | |
Record name | AHR-5955, (S)- | |
Source | FDA Global Substance Registration System (GSRS) | |
URL | https://gsrs.ncats.nih.gov/ginas/app/beta/substances/VL0W61GNK6 | |
Description | The FDA Global Substance Registration System (GSRS) enables the efficient and accurate exchange of information on what substances are in regulated products. Instead of relying on names, which vary across regulatory domains, countries, and regions, the GSRS knowledge base makes it possible for substances to be defined by standardized, scientific descriptions. | |
Explanation | Unless otherwise noted, the contents of the FDA website (www.fda.gov), both text and graphics, are not copyrighted. They are in the public domain and may be republished, reprinted and otherwise used freely by anyone without the need to obtain permission from FDA. Credit to the U.S. Food and Drug Administration as the source is appreciated but not required. | |
Retrosynthesis Analysis
AI-Powered Synthesis Planning: Our tool employs the Template_relevance Pistachio, Template_relevance Bkms_metabolic, Template_relevance Pistachio_ringbreaker, Template_relevance Reaxys, Template_relevance Reaxys_biocatalysis model, leveraging a vast database of chemical reactions to predict feasible synthetic routes.
One-Step Synthesis Focus: Specifically designed for one-step synthesis, it provides concise and direct routes for your target compounds, streamlining the synthesis process.
Accurate Predictions: Utilizing the extensive PISTACHIO, BKMS_METABOLIC, PISTACHIO_RINGBREAKER, REAXYS, REAXYS_BIOCATALYSIS database, our tool offers high-accuracy predictions, reflecting the latest in chemical research and data.
Strategy Settings
Precursor scoring | Relevance Heuristic |
---|---|
Min. plausibility | 0.01 |
Model | Template_relevance |
Template Set | Pistachio/Bkms_metabolic/Pistachio_ringbreaker/Reaxys/Reaxys_biocatalysis |
Top-N result to add to graph | 6 |
Feasible Synthetic Routes
A: Doxapram, when administered intravenously, exhibits a multi-exponential decline in plasma concentration. [] The drug is rapidly absorbed when taken orally in enteric-coated capsules, with a bioavailability of approximately 60%. [] Doxapram undergoes extensive metabolism in the liver, with less than 5% of the administered intravenous dose excreted unchanged in urine within 24 hours. [] One notable metabolite, AHR 5955, exhibits plasma concentrations comparable to doxapram and possesses a similar half-life. []
A: Research suggests that the pharmacokinetic profile of doxapram remains largely consistent between healthy individuals and patients diagnosed with respiratory failure. []
Disclaimer and Information on In-Vitro Research Products
Please be aware that all articles and product information presented on BenchChem are intended solely for informational purposes. The products available for purchase on BenchChem are specifically designed for in-vitro studies, which are conducted outside of living organisms. In-vitro studies, derived from the Latin term "in glass," involve experiments performed in controlled laboratory settings using cells or tissues. It is important to note that these products are not categorized as medicines or drugs, and they have not received approval from the FDA for the prevention, treatment, or cure of any medical condition, ailment, or disease. We must emphasize that any form of bodily introduction of these products into humans or animals is strictly prohibited by law. It is essential to adhere to these guidelines to ensure compliance with legal and ethical standards in research and experimentation.