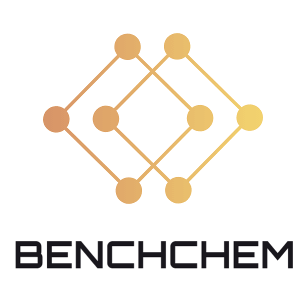
ADB-PINACA
Overview
Description
ADB-PINACA is a cannabinoid designer drug that is an ingredient in some synthetic cannabis products . It is a potent agonist of the CB1 receptor and CB2 receptor with EC50 values of 0.52 nM and 0.88 nM respectively . Like MDMB-FUBINACA, this compound incorporates the unnatural amino acid tert-leucine .
Synthesis Analysis
The synthesis of this compound involves a series of chemical reactions, including the formation of the pyrrolidinopenthiophenone carboxamide moiety, followed by cyclization and functionalization .
Molecular Structure Analysis
This compound has a molecular formula of C19H28N4O2 and a molar mass of 344.459 g·mol−1 . Its IUPAC name is N-(1-Amino-3,3-dimethyl-1-oxo-2-butanyl)-1-pentyl-1H-indazole-3-carboxamide .
Chemical Reactions Analysis
Nineteen major metabolites of this compound were identified in several incubations with cryopreserved human hepatocytes . Major metabolic reactions included pentyl hydroxylation, hydroxylation followed by oxidation (ketone formation), and glucuronidation .
Physical And Chemical Properties Analysis
This compound is a synthetic cannabinoid receptor agonist, characterized by a pyrrolidinopenthiophenone carboxamide moiety . The compound shares structural similarities with other synthetic cannabinoids (SCs), such as ADBICA, AB-FUBINACA, and MDMB-CHMICA .
Scientific Research Applications
Metabolic Analysis and Detection
Metabolism and Detection in Humans : A study by Carlier et al. (2017) focused on the human metabolic fate of ADB-PINACA. They identified major metabolic reactions including hydroxylation, ketone formation, and glucuronidation, providing markers for this compound intake. This research is crucial for distinguishing this compound from similar compounds in clinical and forensic specimens.
Screening in Blood Samples : Tynon et al. (2017) developed and validated a method for screening and confirmation of synthetic cannabinoids like this compound in human whole blood, using liquid chromatography-tandem mass spectrometry. This research aids in forensic investigations and highlights the importance of advanced detection methods for emerging synthetic cannabinoids Tynon et al. (2017).
Pharmacological Studies
Pharmacological Characterization : Banister et al. (2015) synthesized and characterized this compound along with other synthetic cannabinoids. Their study revealed high potency agonist effects on cannabinoid receptors, contributing to our understanding of the pharmacological properties of these substances Banister et al. (2015).
Cannabimimetic Activities : Another study by Schwartz et al. (2015) reported on a cluster of patients experiencing severe symptoms after smoking a product containing this compound. This case cluster was instrumental in understanding the cannabimimetic activities of this compound and its public health implications Schwartz et al. (2015).
Forensic Toxicology
Forensic Case Analysis : Kronstrand et al. (2021) included this compound in their study focusing on the prevalence of synthetic cannabinoids in forensic toxicology cases. They provided insights into the detection and implications of this compound in legal and forensic contexts Kronstrand et al. (2021).
Analysis in Seized Drug Samples : A study by Wouters et al. (2019) examined the activity of carboxy metabolites of synthetic cannabinoids like this compound. Their research is pivotal in understanding the effects of these metabolites in forensic samples Wouters et al. (2019).
Postmortem Blood Analysis : Seither et al. (2020) developed a method for the identification and quantification of 5-fluoro ADB and its metabolites in postmortem blood samples. This research is significant for forensic toxicology in cases involving synthetic cannabinoids Seither et al. (2020).
Legal and Regulatory Aspects
- Controlled Substances Act : A 2016 rule by the Drug Enforcement Administration placed this compound into Schedule I of the Controlled Substances Act, imposing regulatory controls on the handling of this substance. This legal action is crucial for understanding the regulatory status and implications of this compound Federal Register (2016).
Mechanism of Action
ADB-PINACA is a synthetic cannabinoid that has been found in various synthetic cannabis products . It is a potent agonist of the CB1 and CB2 receptors . This article will cover the mechanism of action of this compound, including its primary targets, mode of action, affected biochemical pathways, pharmacokinetics, molecular and cellular effects, and the influence of environmental factors on its action.
Target of Action
The primary targets of this compound are the CB1 and CB2 receptors . These receptors are part of the endocannabinoid system, which plays a crucial role in various physiological processes such as mood, memory, pain sensation, and appetite.
Mode of Action
This compound acts as a potent agonist at the CB1 and CB2 receptors . Agonists are substances that bind to receptors and activate them to produce a biological response. In this case, this compound mimics the action of endogenous cannabinoids by binding to these receptors and activating them.
Biochemical Pathways
Upon activation of the CB1 and CB2 receptors, this compound triggers several downstream effects in the endocannabinoid systemIt is known that the activation of cb1 receptors can inhibit the release of various neurotransmitters, thereby affecting neuronal excitability and synaptic plasticity .
Pharmacokinetics
It has been found that major metabolic reactions of this compound include pentyl hydroxylation, hydroxylation followed by oxidation (ketone formation), and glucuronidation . These metabolic reactions can affect the compound’s bioavailability and duration of action.
Result of Action
The activation of CB1 and CB2 receptors by this compound can lead to various molecular and cellular effects. These effects can mimic those of Δ9-tetrahydrocannabinol (THC), the main psychoactive constituent of cannabis . This compound has been linked to several severe intoxication cases , indicating that its effects can be more potent and unpredictable than those of natural cannabinoids.
Action Environment
Environmental factors can influence the action, efficacy, and stability of this compound. For instance, factors such as temperature and pH can affect the compound’s stability and its interaction with the CB1 and CB2 receptors. Furthermore, individual factors, including a person’s genetics, overall health, and use of other substances, can influence how this compound is metabolized and how it affects the body .
Safety and Hazards
Future Directions
Biochemical Analysis
Biochemical Properties
ADB-PINACA plays a crucial role in biochemical reactions by interacting with cannabinoid receptors. It is a potent agonist of the CB1 and CB2 receptors, with effective concentration (EC50) values of 0.52 nanomolar and 0.88 nanomolar, respectively . These interactions are characterized by the binding of this compound to the active sites of these receptors, leading to the activation of downstream signaling pathways. The compound’s structure, which includes a 1-amino-3,3-dimethyl-1-oxobutan-2-yl group and a 1-pentyl-1H-indazole-3-carboxamide group, facilitates its high affinity for these receptors .
Cellular Effects
This compound exerts significant effects on various types of cells and cellular processes. Upon binding to CB1 and CB2 receptors, this compound influences cell signaling pathways, including those involved in neurotransmitter release, gene expression, and cellular metabolism . The activation of these receptors by this compound can lead to altered neuronal activity, changes in gene transcription, and modulation of metabolic processes. These effects are particularly pronounced in neuronal cells, where this compound can induce psychoactive effects similar to those of delta-9-tetrahydrocannabinol (THC), the primary psychoactive component of cannabis .
Molecular Mechanism
The molecular mechanism of this compound involves its binding to cannabinoid receptors, leading to the activation of G-protein coupled receptor (GPCR) signaling pathways. Upon binding to CB1 and CB2 receptors, this compound induces conformational changes in these receptors, which in turn activate associated G-proteins . This activation triggers a cascade of intracellular events, including the inhibition of adenylate cyclase, reduction of cyclic adenosine monophosphate (cAMP) levels, and modulation of ion channel activity. Additionally, this compound can influence gene expression by activating transcription factors such as CREB (cAMP response element-binding protein), leading to changes in the expression of genes involved in various cellular functions .
Temporal Effects in Laboratory Settings
In laboratory settings, the effects of this compound have been observed to change over time. The compound exhibits rapid onset of action, with effects typically appearing within minutes of exposure . The duration of these effects can vary depending on the concentration and exposure time. This compound is relatively stable under laboratory conditions, but it can undergo degradation over extended periods, leading to a decrease in its potency . Long-term exposure to this compound in in vitro and in vivo studies has shown potential for persistent alterations in cellular function, including changes in receptor sensitivity and gene expression .
Dosage Effects in Animal Models
The effects of this compound in animal models vary with different dosages. At low doses, this compound can induce mild psychoactive effects, while higher doses can lead to severe intoxication and adverse effects . In mice, dose-dependent convulsant effects have been observed, which are mediated by the activation of CB1 receptors . These convulsant effects can be blocked by pretreatment with CB1 receptor antagonists, indicating the specificity of this compound’s action on these receptors . At high doses, this compound can also induce toxic effects, including respiratory depression and cardiovascular complications .
Metabolic Pathways
This compound undergoes extensive metabolism in the body, primarily through hydroxylation, oxidation, and glucuronidation . In human hepatocytes, nineteen major metabolites of this compound have been identified, including hydroxylated and ketone derivatives . These metabolic reactions are facilitated by enzymes such as cytochrome P450s, which play a key role in the biotransformation of this compound . The metabolic pathways of this compound also involve the formation of glucuronide conjugates, which enhance the compound’s solubility and facilitate its excretion .
Transport and Distribution
Within cells and tissues, this compound is transported and distributed through interactions with various transporters and binding proteins . The compound’s lipophilic nature allows it to readily cross cell membranes and accumulate in lipid-rich tissues . This compound can also bind to plasma proteins, which influences its distribution and bioavailability . The localization of this compound within specific tissues, such as the brain, contributes to its potent psychoactive effects .
Subcellular Localization
The subcellular localization of this compound is primarily within the cell membrane, where it interacts with cannabinoid receptors . The compound’s lipophilic properties facilitate its incorporation into the lipid bilayer of the cell membrane, allowing it to access and activate CB1 and CB2 receptors . Additionally, this compound may undergo post-translational modifications that influence its targeting to specific cellular compartments . These modifications can affect the compound’s activity and function, contributing to its overall pharmacological profile .
Properties
{ "Design of the Synthesis Pathway": "The synthesis pathway of ADB-PINACA involves the reaction of a substituted indazole with a substituted naphthalene carboxylic acid chloride in the presence of a base.", "Starting Materials": [ "Substituted indazole", "Substituted naphthalene carboxylic acid chloride", "Base" ], "Reaction": [ "The substituted indazole is dissolved in a suitable solvent and the base is added to the solution.", "The substituted naphthalene carboxylic acid chloride is added dropwise to the solution while stirring.", "The reaction mixture is heated to reflux for several hours.", "The reaction mixture is cooled and the product is extracted with a suitable solvent.", "The solvent is evaporated to obtain the crude product.", "The crude product is purified by column chromatography to obtain pure ADB-PINACA." ] } | |
1633766-73-0 | |
Molecular Formula |
C19H28N4O2 |
Molecular Weight |
344.5 g/mol |
IUPAC Name |
N-(1-amino-3,3-dimethyl-1-oxobutan-2-yl)-1-pentylindazole-3-carboxamide |
InChI |
InChI=1S/C19H28N4O2/c1-5-6-9-12-23-14-11-8-7-10-13(14)15(22-23)18(25)21-16(17(20)24)19(2,3)4/h7-8,10-11,16H,5-6,9,12H2,1-4H3,(H2,20,24)(H,21,25) |
InChI Key |
FWTARAXQGJRQKN-UHFFFAOYSA-N |
SMILES |
O=C(C1=NN(CCCCC)C2=C1C=CC=C2)NC(C(C)(C)C)C(N)=O |
Canonical SMILES |
CCCCCN1C2=CC=CC=C2C(=N1)C(=O)NC(C(=O)N)C(C)(C)C |
Appearance |
Solid powder |
Purity |
>98% (or refer to the Certificate of Analysis) |
shelf_life |
>3 years if stored properly |
solubility |
Soluble in DMSO |
storage |
Dry, dark and at 0 - 4 C for short term (days to weeks) or -20 C for long term (months to years). |
synonyms |
ADB-PINACA; UNII-U1Q4F41C9U |
Origin of Product |
United States |
Retrosynthesis Analysis
AI-Powered Synthesis Planning: Our tool employs the Template_relevance Pistachio, Template_relevance Bkms_metabolic, Template_relevance Pistachio_ringbreaker, Template_relevance Reaxys, Template_relevance Reaxys_biocatalysis model, leveraging a vast database of chemical reactions to predict feasible synthetic routes.
One-Step Synthesis Focus: Specifically designed for one-step synthesis, it provides concise and direct routes for your target compounds, streamlining the synthesis process.
Accurate Predictions: Utilizing the extensive PISTACHIO, BKMS_METABOLIC, PISTACHIO_RINGBREAKER, REAXYS, REAXYS_BIOCATALYSIS database, our tool offers high-accuracy predictions, reflecting the latest in chemical research and data.
Strategy Settings
Precursor scoring | Relevance Heuristic |
---|---|
Min. plausibility | 0.01 |
Model | Template_relevance |
Template Set | Pistachio/Bkms_metabolic/Pistachio_ringbreaker/Reaxys/Reaxys_biocatalysis |
Top-N result to add to graph | 6 |
Feasible Synthetic Routes
Disclaimer and Information on In-Vitro Research Products
Please be aware that all articles and product information presented on BenchChem are intended solely for informational purposes. The products available for purchase on BenchChem are specifically designed for in-vitro studies, which are conducted outside of living organisms. In-vitro studies, derived from the Latin term "in glass," involve experiments performed in controlled laboratory settings using cells or tissues. It is important to note that these products are not categorized as medicines or drugs, and they have not received approval from the FDA for the prevention, treatment, or cure of any medical condition, ailment, or disease. We must emphasize that any form of bodily introduction of these products into humans or animals is strictly prohibited by law. It is essential to adhere to these guidelines to ensure compliance with legal and ethical standards in research and experimentation.