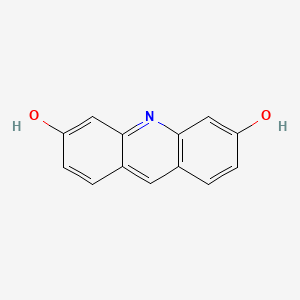
Acridine-3,6-diol
Overview
Description
Acridine-3,6-diol is a derivative of acridine, a heterocyclic organic compound containing nitrogen. Acridine derivatives are known for their broad range of pharmaceutical properties, including anti-inflammatory, anticancer, antimicrobial, antitubercular, antiparasitic, antimalarial, antiviral, and fungicidal activities . This compound, specifically, is characterized by the presence of hydroxyl groups at the 3rd and 6th positions of the acridine ring, which may contribute to its unique chemical and biological properties.
Preparation Methods
Synthetic Routes and Reaction Conditions: The synthesis of acridine-3,6-diol typically involves the hydroxylation of acridine derivatives. One common method is the direct hydroxylation of acridine using strong oxidizing agents under controlled conditions.
Industrial Production Methods: Industrial production of this compound may involve multi-step synthesis starting from readily available acridine derivatives. The process may include steps such as nitration, reduction, and subsequent hydroxylation. The reaction conditions are optimized to ensure high yield and purity of the final product .
Chemical Reactions Analysis
Types of Reactions: Acridine-3,6-diol can undergo various chemical reactions, including:
Oxidation: The hydroxyl groups can be oxidized to form quinone derivatives.
Reduction: The compound can be reduced to form dihydro derivatives.
Substitution: The hydroxyl groups can be substituted with other functional groups, such as halogens or alkyl groups.
Common Reagents and Conditions:
Oxidation: Common oxidizing agents include hydrogen peroxide and potassium permanganate.
Reduction: Reducing agents such as sodium borohydride or lithium aluminum hydride can be used.
Substitution: Halogenating agents like thionyl chloride or alkylating agents like methyl iodide are commonly employed.
Major Products:
Oxidation: Quinone derivatives.
Reduction: Dihydro derivatives.
Substitution: Halogenated or alkylated acridine derivatives.
Scientific Research Applications
Acridine-3,6-diol has a wide range of applications in scientific research:
Medicine: Explored for its anticancer, antimicrobial, and antiviral properties.
Industry: Utilized in the production of dyes and pigments due to its vibrant color and stability.
Mechanism of Action
The mechanism of action of acridine-3,6-diol primarily involves its ability to intercalate into DNA. This intercalation disrupts the normal function of DNA and inhibits the activity of enzymes such as topoisomerase and telomerase. By interfering with these enzymes, this compound can inhibit the replication and transcription of DNA, leading to the death of cancer cells . Additionally, the hydroxyl groups may enhance its binding affinity and specificity for certain molecular targets .
Comparison with Similar Compounds
Proflavine: Another acridine derivative with similar antibacterial and anticancer activities.
Amsacrine: An acridine derivative used as an anticancer agent, particularly in the treatment of leukemia.
Uniqueness of Acridine-3,6-diol: this compound is unique due to the presence of hydroxyl groups at specific positions, which may enhance its chemical reactivity and biological activity. This structural feature allows for unique interactions with DNA and other biomolecules, potentially leading to more effective therapeutic applications .
Properties
IUPAC Name |
acridine-3,6-diol | |
---|---|---|
Source | PubChem | |
URL | https://pubchem.ncbi.nlm.nih.gov | |
Description | Data deposited in or computed by PubChem | |
InChI |
InChI=1S/C13H9NO2/c15-10-3-1-8-5-9-2-4-11(16)7-13(9)14-12(8)6-10/h1-7,15-16H | |
Source | PubChem | |
URL | https://pubchem.ncbi.nlm.nih.gov | |
Description | Data deposited in or computed by PubChem | |
InChI Key |
LXFYIIKDJZNGST-UHFFFAOYSA-N | |
Source | PubChem | |
URL | https://pubchem.ncbi.nlm.nih.gov | |
Description | Data deposited in or computed by PubChem | |
Canonical SMILES |
C1=CC(=CC2=NC3=C(C=CC(=C3)O)C=C21)O | |
Source | PubChem | |
URL | https://pubchem.ncbi.nlm.nih.gov | |
Description | Data deposited in or computed by PubChem | |
Molecular Formula |
C13H9NO2 | |
Source | PubChem | |
URL | https://pubchem.ncbi.nlm.nih.gov | |
Description | Data deposited in or computed by PubChem | |
DSSTOX Substance ID |
DTXSID60962913 | |
Record name | 6-Hydroxyacridin-3(10H)-one | |
Source | EPA DSSTox | |
URL | https://comptox.epa.gov/dashboard/DTXSID60962913 | |
Description | DSSTox provides a high quality public chemistry resource for supporting improved predictive toxicology. | |
Molecular Weight |
211.22 g/mol | |
Source | PubChem | |
URL | https://pubchem.ncbi.nlm.nih.gov | |
Description | Data deposited in or computed by PubChem | |
CAS No. |
43129-74-4 | |
Record name | 3,6-Acridinediol | |
Source | CAS Common Chemistry | |
URL | https://commonchemistry.cas.org/detail?cas_rn=43129-74-4 | |
Description | CAS Common Chemistry is an open community resource for accessing chemical information. Nearly 500,000 chemical substances from CAS REGISTRY cover areas of community interest, including common and frequently regulated chemicals, and those relevant to high school and undergraduate chemistry classes. This chemical information, curated by our expert scientists, is provided in alignment with our mission as a division of the American Chemical Society. | |
Explanation | The data from CAS Common Chemistry is provided under a CC-BY-NC 4.0 license, unless otherwise stated. | |
Record name | Acridine-3,6-diol | |
Source | ChemIDplus | |
URL | https://pubchem.ncbi.nlm.nih.gov/substance/?source=chemidplus&sourceid=0043129744 | |
Description | ChemIDplus is a free, web search system that provides access to the structure and nomenclature authority files used for the identification of chemical substances cited in National Library of Medicine (NLM) databases, including the TOXNET system. | |
Record name | 6-Hydroxyacridin-3(10H)-one | |
Source | EPA DSSTox | |
URL | https://comptox.epa.gov/dashboard/DTXSID60962913 | |
Description | DSSTox provides a high quality public chemistry resource for supporting improved predictive toxicology. | |
Record name | Acridine-3,6-diol | |
Source | European Chemicals Agency (ECHA) | |
URL | https://echa.europa.eu/substance-information/-/substanceinfo/100.050.991 | |
Description | The European Chemicals Agency (ECHA) is an agency of the European Union which is the driving force among regulatory authorities in implementing the EU's groundbreaking chemicals legislation for the benefit of human health and the environment as well as for innovation and competitiveness. | |
Explanation | Use of the information, documents and data from the ECHA website is subject to the terms and conditions of this Legal Notice, and subject to other binding limitations provided for under applicable law, the information, documents and data made available on the ECHA website may be reproduced, distributed and/or used, totally or in part, for non-commercial purposes provided that ECHA is acknowledged as the source: "Source: European Chemicals Agency, http://echa.europa.eu/". Such acknowledgement must be included in each copy of the material. ECHA permits and encourages organisations and individuals to create links to the ECHA website under the following cumulative conditions: Links can only be made to webpages that provide a link to the Legal Notice page. | |
Disclaimer and Information on In-Vitro Research Products
Please be aware that all articles and product information presented on BenchChem are intended solely for informational purposes. The products available for purchase on BenchChem are specifically designed for in-vitro studies, which are conducted outside of living organisms. In-vitro studies, derived from the Latin term "in glass," involve experiments performed in controlled laboratory settings using cells or tissues. It is important to note that these products are not categorized as medicines or drugs, and they have not received approval from the FDA for the prevention, treatment, or cure of any medical condition, ailment, or disease. We must emphasize that any form of bodily introduction of these products into humans or animals is strictly prohibited by law. It is essential to adhere to these guidelines to ensure compliance with legal and ethical standards in research and experimentation.