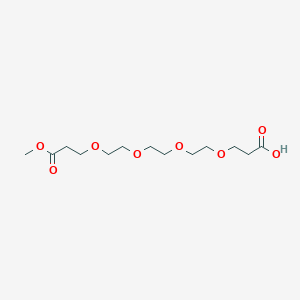
Acid-PEG4-mono-methyl ester
Overview
Description
Acid-PEG4-mono-methyl ester: is a polyethylene glycol (PEG) derivative with a carboxylic acid and methyl ester group. This compound is known for its hydrophilic properties, which increase its solubility in aqueous media. The carboxylic acid group can react with primary amines to form stable amide bonds, making it a valuable linker in various chemical and biological applications .
Mechanism of Action
Target of Action
Acid-PEG4-mono-methyl ester is primarily used as a PROTAC linker . PROTACs (Proteolysis-Targeting Chimeras) are a class of bifunctional molecules that recruit an E3 ubiquitin ligase to a target protein, leading to the target’s degradation . The primary targets of this compound, therefore, are the proteins that are intended to be degraded by the PROTAC.
Mode of Action
This compound acts as a bridge in PROTACs, connecting a ligand for an E3 ubiquitin ligase with a ligand for the target protein . This allows the PROTAC to bring the E3 ligase and the target protein into close proximity, facilitating the transfer of ubiquitin from the E3 ligase to the target. The ubiquitinated target protein is then recognized by the proteasome, an intracellular protein complex, and is degraded .
Biochemical Pathways
The key biochemical pathway involved in the action of this compound is the ubiquitin-proteasome system . This system is responsible for the degradation of most proteins in the cytoplasm and nucleus of eukaryotic cells. By facilitating the ubiquitination and subsequent degradation of target proteins, this compound (via PROTACs) can influence numerous cellular processes, depending on the function of the target protein.
Pharmacokinetics
It’s known that the compound is a peg (polyethylene glycol) derivative . PEGylation, or the addition of PEG chains to molecules, is a common strategy to improve the pharmacokinetics of drugs. It can increase solubility, stability, and half-life, and decrease immunogenicity and proteolytic degradation .
Result of Action
The result of this compound’s action is the degradation of the target protein . This can have various effects at the molecular and cellular levels, depending on the role of the target protein. For example, if the target protein is a key player in a disease pathway, its degradation could potentially ameliorate the disease.
Action Environment
The action of this compound is influenced by the intracellular environment. For instance, the efficiency of the ubiquitin-proteasome system can be affected by various factors, including pH, temperature, and the presence of other proteins . Moreover, the compound’s methyl ester group can be hydrolyzed under strong basic conditions , which could potentially affect its stability and efficacy.
Biochemical Analysis
Biochemical Properties
Acid-PEG4-mono-methyl ester plays a role in biochemical reactions as a non-cleavable linker for bio-conjugation . It contains an Azide group and a COOR/Ester group linked through a linear PEG chain . The carboxylic acid can react with primary amines in the presence of EDC and HATU to form stable amide bonds .
Temporal Effects in Laboratory Settings
The temporal effects of this compound in laboratory settings are not well-documented in the literature. It is known that this compound is stable and can be stored at -20°C .
Preparation Methods
Synthetic Routes and Reaction Conditions:
Nucleophilic Displacement: One common method involves the nucleophilic displacement of PEG-tosylate or PEG-mesylate with a suitable nucleophile.
Reductive Amination: Another method includes the reductive amination of PEG-aldehyde or PEG-amine.
Industrial Production Methods: Industrial production of Acid-PEG4-mono-methyl ester typically involves large-scale esterification processes, where the PEG derivative is reacted with fatty acid chlorides under controlled conditions to ensure high yield and purity .
Chemical Reactions Analysis
Types of Reactions:
Common Reagents and Conditions:
Hydrolysis: Strong bases such as sodium hydroxide (NaOH) or potassium hydroxide (KOH).
Amide Bond Formation: EDC and HATU as coupling agents, often in the presence of a base like N,N-diisopropylethylamine (DIPEA).
Major Products:
Hydrolysis: Produces the corresponding carboxylic acid and methanol.
Amide Bond Formation: Produces stable amide bonds, linking the PEG derivative to other molecules.
Scientific Research Applications
Comparison with Similar Compounds
- Acid-PEG1-t-butyl ester
- Bis-PEG12-acid
- S-acetyl-PEG3-acid
- Acid-PEG12-NHS ester
- Acid-PEG10-NHS ester
- Acid-PEG5-NHS ester
- Acid-PEG25-NHS ester
- Acid-PEG5-mono-methyl ester
- Acid-PEG6-mono-methyl ester
- Acid-PEG3-t-butyl ester
- Acid-PEG9-NHS ester
- Acid-PEG13-NHS ester
- Acid-PEG4-t-butyl ester
- Acid-PEG4-Sulfone-PEG4-Acid
- Acid-PEG4-S-PEG4-Acid
Uniqueness:
- Acid-PEG4-mono-methyl ester is unique due to its specific combination of a carboxylic acid and a methyl ester group, which provides a balance of reactivity and stability. Its hydrophilic PEG linker enhances solubility in aqueous media, making it particularly useful in biological and medical applications .
Properties
IUPAC Name |
3-[2-[2-[2-(3-methoxy-3-oxopropoxy)ethoxy]ethoxy]ethoxy]propanoic acid | |
---|---|---|
Source | PubChem | |
URL | https://pubchem.ncbi.nlm.nih.gov | |
Description | Data deposited in or computed by PubChem | |
InChI |
InChI=1S/C13H24O8/c1-17-13(16)3-5-19-7-9-21-11-10-20-8-6-18-4-2-12(14)15/h2-11H2,1H3,(H,14,15) | |
Source | PubChem | |
URL | https://pubchem.ncbi.nlm.nih.gov | |
Description | Data deposited in or computed by PubChem | |
InChI Key |
MOGNNSWXCSXEEM-UHFFFAOYSA-N | |
Source | PubChem | |
URL | https://pubchem.ncbi.nlm.nih.gov | |
Description | Data deposited in or computed by PubChem | |
Canonical SMILES |
COC(=O)CCOCCOCCOCCOCCC(=O)O | |
Source | PubChem | |
URL | https://pubchem.ncbi.nlm.nih.gov | |
Description | Data deposited in or computed by PubChem | |
Molecular Formula |
C13H24O8 | |
Source | PubChem | |
URL | https://pubchem.ncbi.nlm.nih.gov | |
Description | Data deposited in or computed by PubChem | |
Molecular Weight |
308.32 g/mol | |
Source | PubChem | |
URL | https://pubchem.ncbi.nlm.nih.gov | |
Description | Data deposited in or computed by PubChem | |
Retrosynthesis Analysis
AI-Powered Synthesis Planning: Our tool employs the Template_relevance Pistachio, Template_relevance Bkms_metabolic, Template_relevance Pistachio_ringbreaker, Template_relevance Reaxys, Template_relevance Reaxys_biocatalysis model, leveraging a vast database of chemical reactions to predict feasible synthetic routes.
One-Step Synthesis Focus: Specifically designed for one-step synthesis, it provides concise and direct routes for your target compounds, streamlining the synthesis process.
Accurate Predictions: Utilizing the extensive PISTACHIO, BKMS_METABOLIC, PISTACHIO_RINGBREAKER, REAXYS, REAXYS_BIOCATALYSIS database, our tool offers high-accuracy predictions, reflecting the latest in chemical research and data.
Strategy Settings
Precursor scoring | Relevance Heuristic |
---|---|
Min. plausibility | 0.01 |
Model | Template_relevance |
Template Set | Pistachio/Bkms_metabolic/Pistachio_ringbreaker/Reaxys/Reaxys_biocatalysis |
Top-N result to add to graph | 6 |
Feasible Synthetic Routes
Disclaimer and Information on In-Vitro Research Products
Please be aware that all articles and product information presented on BenchChem are intended solely for informational purposes. The products available for purchase on BenchChem are specifically designed for in-vitro studies, which are conducted outside of living organisms. In-vitro studies, derived from the Latin term "in glass," involve experiments performed in controlled laboratory settings using cells or tissues. It is important to note that these products are not categorized as medicines or drugs, and they have not received approval from the FDA for the prevention, treatment, or cure of any medical condition, ailment, or disease. We must emphasize that any form of bodily introduction of these products into humans or animals is strictly prohibited by law. It is essential to adhere to these guidelines to ensure compliance with legal and ethical standards in research and experimentation.