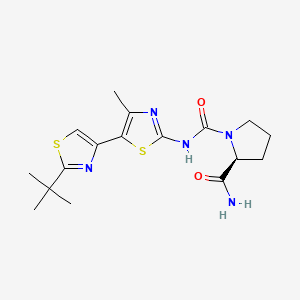
A66
Overview
Description
A66 is a highly specific and selective inhibitor of the enzyme phosphoinositide 3-kinase catalytic subunit alpha (p110α). It has an inhibitory concentration (IC50) of 32 nanomolar for the wild-type p110α, 30 nanomolar for the p110α E545K mutant, and 43 nanomolar for the p110α H1047R mutant . This compound is primarily used in scientific research to study the phosphoinositide 3-kinase (PI3K) signaling pathway, which is involved in various cellular processes such as growth, proliferation, and survival.
Mechanism of Action
Mode of Action
A66 interacts with its target, the p110α subunit of PI3K, by inhibiting its activity . This inhibition is highly specific and selective, with an IC50 of 32 nM . This compound also potently inhibits the oncogenic forms of p110α, such as p110α E545K and p110α H1047R .
Biochemical Pathways
The inhibition of the p110α subunit of PI3K by this compound affects the PI3K/Akt/mTOR signaling pathway . This pathway is involved in many cellular processes including cell cycle progression, cell survival, and angiogenesis . By inhibiting the p110α subunit, this compound disrupts this pathway, potentially leading to reduced cell proliferation and survival .
Result of Action
The molecular and cellular effects of this compound’s action primarily involve the inhibition of the PI3K/Akt/mTOR signaling pathway . This can lead to reduced cell proliferation and survival . In addition, this compound has been shown to effectively reduce the phosphorylation of Akt/PKB and p70 S6 kinase, inhibiting tumor cell growth .
Biochemical Analysis
Biochemical Properties
A66 is a potent and selective inhibitor of the p110α isoform of class I phosphoinositide 3-kinases (PI3Ks) . It interacts with the p110α isoform, exhibiting over 100-fold selectivity for p110α over other PI3K isoforms . This interaction is crucial in the regulation of various biochemical reactions within the cell .
Cellular Effects
In cellular contexts, this compound has been shown to have significant effects on various types of cells and cellular processes. It influences cell function by modulating the PI3K/Akt signaling pathway . This compound has been shown to inhibit the activation of Akt, a key player in cellular survival and metabolism pathways .
Molecular Mechanism
The molecular mechanism of this compound involves its binding to the p110α isoform of PI3Ks, leading to the inhibition of the kinase activity of this enzyme . This results in the downregulation of the PI3K/Akt signaling pathway, affecting various downstream processes such as cell survival, proliferation, and metabolism .
Metabolic Pathways
This compound is involved in the PI3K/Akt signaling pathway, a critical metabolic pathway in cells . This pathway is involved in a variety of cellular processes, including cell survival, growth, proliferation, and metabolism .
Preparation Methods
Synthetic Routes and Reaction Conditions
The synthesis of A66 involves multiple steps, starting from commercially available starting materials. The key steps include the formation of the bithiazole core and the subsequent functionalization to introduce the pyrrolidine and carboxamide groups. The reaction conditions typically involve the use of organic solvents, catalysts, and specific temperature controls to ensure high yield and purity .
Industrial Production Methods
Industrial production of this compound follows similar synthetic routes but on a larger scale. The process is optimized for efficiency and cost-effectiveness, often involving continuous flow reactors and automated systems to control reaction parameters. Purification is achieved through techniques such as crystallization, chromatography, and recrystallization to obtain the compound with high purity .
Chemical Reactions Analysis
Types of Reactions
A66 undergoes various chemical reactions, including:
Oxidation: this compound can be oxidized under specific conditions to form oxidized derivatives.
Reduction: Reduction reactions can modify the functional groups on this compound, potentially altering its inhibitory activity.
Common Reagents and Conditions
Oxidation: Common oxidizing agents include hydrogen peroxide and potassium permanganate.
Reduction: Reducing agents such as sodium borohydride and lithium aluminum hydride are used.
Substitution: Substitution reactions often involve nucleophiles like amines or thiols under basic or acidic conditions.
Major Products Formed
The major products formed from these reactions include various analogs of this compound with modified functional groups. These analogs are often studied to understand the structure-activity relationship and to develop more potent inhibitors .
Scientific Research Applications
A66 is widely used in scientific research due to its specificity and potency as a p110α inhibitor. Some key applications include:
Chemistry: this compound is used to study the PI3K signaling pathway and its role in various chemical reactions and processes.
Biology: In biological research, this compound helps in understanding the role of PI3K in cell growth, proliferation, and survival.
Medicine: this compound has potential therapeutic applications in cancer treatment, as PI3K signaling is often dysregulated in cancer cells.
Comparison with Similar Compounds
Similar Compounds
PIK-75: Another p110α inhibitor with a different chemical structure and lower specificity compared to A66.
BEZ-235: A dual PI3K/mTOR inhibitor with broader activity against multiple PI3K isoforms.
GDC-0941: A selective inhibitor of class I PI3K isoforms, including p110α
Uniqueness of this compound
This compound is unique due to its high specificity and selectivity for the p110α subunit of PI3K. Unlike other inhibitors, this compound exhibits minimal cross-reactivity with other PI3K isoforms and related kinases. This specificity makes this compound a valuable tool for studying the PI3K pathway and its role in various cellular processes .
Properties
IUPAC Name |
(2S)-1-N-[5-(2-tert-butyl-1,3-thiazol-4-yl)-4-methyl-1,3-thiazol-2-yl]pyrrolidine-1,2-dicarboxamide | |
---|---|---|
Source | PubChem | |
URL | https://pubchem.ncbi.nlm.nih.gov | |
Description | Data deposited in or computed by PubChem | |
InChI |
InChI=1S/C17H23N5O2S2/c1-9-12(10-8-25-14(20-10)17(2,3)4)26-15(19-9)21-16(24)22-7-5-6-11(22)13(18)23/h8,11H,5-7H2,1-4H3,(H2,18,23)(H,19,21,24)/t11-/m0/s1 | |
Source | PubChem | |
URL | https://pubchem.ncbi.nlm.nih.gov | |
Description | Data deposited in or computed by PubChem | |
InChI Key |
HBPXWEPKNBHKAX-NSHDSACASA-N | |
Source | PubChem | |
URL | https://pubchem.ncbi.nlm.nih.gov | |
Description | Data deposited in or computed by PubChem | |
Canonical SMILES |
CC1=C(SC(=N1)NC(=O)N2CCCC2C(=O)N)C3=CSC(=N3)C(C)(C)C | |
Source | PubChem | |
URL | https://pubchem.ncbi.nlm.nih.gov | |
Description | Data deposited in or computed by PubChem | |
Isomeric SMILES |
CC1=C(SC(=N1)NC(=O)N2CCC[C@H]2C(=O)N)C3=CSC(=N3)C(C)(C)C | |
Source | PubChem | |
URL | https://pubchem.ncbi.nlm.nih.gov | |
Description | Data deposited in or computed by PubChem | |
Molecular Formula |
C17H23N5O2S2 | |
Source | PubChem | |
URL | https://pubchem.ncbi.nlm.nih.gov | |
Description | Data deposited in or computed by PubChem | |
DSSTOX Substance ID |
DTXSID80655299 | |
Record name | (2S)-N~1~-(2-tert-Butyl-4'-methyl[4,5'-bi-1,3-thiazol]-2'-yl)pyrrolidine-1,2-dicarboxamide | |
Source | EPA DSSTox | |
URL | https://comptox.epa.gov/dashboard/DTXSID80655299 | |
Description | DSSTox provides a high quality public chemistry resource for supporting improved predictive toxicology. | |
Molecular Weight |
393.5 g/mol | |
Source | PubChem | |
URL | https://pubchem.ncbi.nlm.nih.gov | |
Description | Data deposited in or computed by PubChem | |
CAS No. |
1166227-08-2 | |
Record name | (2S)-N~1~-(2-tert-Butyl-4'-methyl[4,5'-bi-1,3-thiazol]-2'-yl)pyrrolidine-1,2-dicarboxamide | |
Source | EPA DSSTox | |
URL | https://comptox.epa.gov/dashboard/DTXSID80655299 | |
Description | DSSTox provides a high quality public chemistry resource for supporting improved predictive toxicology. | |
Retrosynthesis Analysis
AI-Powered Synthesis Planning: Our tool employs the Template_relevance Pistachio, Template_relevance Bkms_metabolic, Template_relevance Pistachio_ringbreaker, Template_relevance Reaxys, Template_relevance Reaxys_biocatalysis model, leveraging a vast database of chemical reactions to predict feasible synthetic routes.
One-Step Synthesis Focus: Specifically designed for one-step synthesis, it provides concise and direct routes for your target compounds, streamlining the synthesis process.
Accurate Predictions: Utilizing the extensive PISTACHIO, BKMS_METABOLIC, PISTACHIO_RINGBREAKER, REAXYS, REAXYS_BIOCATALYSIS database, our tool offers high-accuracy predictions, reflecting the latest in chemical research and data.
Strategy Settings
Precursor scoring | Relevance Heuristic |
---|---|
Min. plausibility | 0.01 |
Model | Template_relevance |
Template Set | Pistachio/Bkms_metabolic/Pistachio_ringbreaker/Reaxys/Reaxys_biocatalysis |
Top-N result to add to graph | 6 |
Feasible Synthetic Routes
Disclaimer and Information on In-Vitro Research Products
Please be aware that all articles and product information presented on BenchChem are intended solely for informational purposes. The products available for purchase on BenchChem are specifically designed for in-vitro studies, which are conducted outside of living organisms. In-vitro studies, derived from the Latin term "in glass," involve experiments performed in controlled laboratory settings using cells or tissues. It is important to note that these products are not categorized as medicines or drugs, and they have not received approval from the FDA for the prevention, treatment, or cure of any medical condition, ailment, or disease. We must emphasize that any form of bodily introduction of these products into humans or animals is strictly prohibited by law. It is essential to adhere to these guidelines to ensure compliance with legal and ethical standards in research and experimentation.