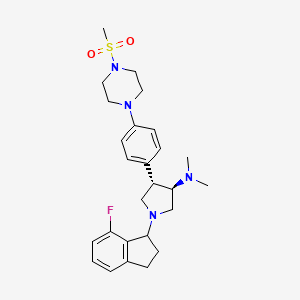
A-395
Overview
Description
Cloprostenol is a synthetic analogue of prostaglandin F2α, a naturally occurring prostaglandin. It is primarily used in veterinary medicine as a potent luteolytic agent, meaning it induces the regression of the corpus luteum, a temporary endocrine structure in female mammals . This effect is utilized to control estrus cycles and induce abortion in animals .
Mechanism of Action
Target of Action
A-395, also known as (3R,4S)-1-(7-fluoro-2,3-dihydro-1H-inden-1-yl)-N,N-dimethyl-4-(4-(4-(methylsulfonyl)piperazin-1-yl)phenyl)pyrrolidin-3-amine, is a potent and selective antagonist of the Polycomb Repressive Complex 2 (PRC2) . The primary targets of this compound are the proteins within the PRC2 complex, specifically EZH2, EED, and SUZ12 .
Mode of Action
This compound binds to EED in the H3K27me3-binding pocket, thereby preventing allosteric activation of the catalytic activity of PRC2 . It competes for H3K27me3 peptide binding to EED, with an IC50 of 7 nM . This interaction disrupts the function of the PRC2 complex, leading to a reduction in the methylation of H3K27 .
Biochemical Pathways
The primary biochemical pathway affected by this compound is the histone methylation pathway . By inhibiting the PRC2 complex, this compound reduces the methylation of H3K27 . This can lead to changes in gene expression, as H3K27 methylation is a key epigenetic modification associated with gene repression .
Pharmacokinetics
It’s known that this compound has potent in vitro activity, with a ki of 04 nM for binding to EED and an IC50 of 34 nM for inhibiting the PRC2 complex .
Result of Action
The primary result of this compound’s action is the inhibition of the PRC2 complex and the subsequent reduction in H3K27 methylation . This can lead to changes in gene expression, potentially impacting cellular processes such as cell proliferation .
Biochemical Analysis
Biochemical Properties
A-395 plays a crucial role in biochemical reactions by binding to the EED component of the PRC2 complex with a Ki value of 0.4 nM. This binding inhibits the PRC2 complex, which is responsible for the methylation of histone H3K27. The inhibition of PRC2 by this compound results in an IC50 value of 34 nM for the methylation of H3K27, demonstrating its potency and selectivity. The compound exhibits greater than 100-fold selectivity over other histone methyltransferases and non-epigenetic targets .
Cellular Effects
This compound has significant effects on various types of cells and cellular processes. In cellular assays, this compound inhibits the PRC2 complex, leading to the inhibition of H3K27me3 formation with an IC50 value of 90 nM in the RD rhabdoid tumor cell line. This inhibition impacts cell signaling pathways, gene expression, and cellular metabolism by preventing the transcriptional repression mediated by PRC2 .
Molecular Mechanism
The molecular mechanism of this compound involves its binding to the EED component of the PRC2 complex. This binding inhibits the enzymatic activity of PRC2, preventing the methylation of histone H3K27. As a result, the transcriptional repression of target genes is alleviated, leading to changes in gene expression. This compound’s selective inhibition of PRC2 highlights its potential as a valuable tool for studying the role of PRC2 in various biological processes .
Temporal Effects in Laboratory Settings
In laboratory settings, the effects of this compound have been observed to change over time. The compound’s stability and degradation have been studied to ensure its efficacy in long-term experiments. This compound has shown consistent inhibition of PRC2 activity over extended periods, making it a reliable tool for in vitro and in vivo studies. Long-term effects on cellular function have also been noted, with sustained inhibition of H3K27me3 formation observed in treated cells .
Dosage Effects in Animal Models
The effects of this compound vary with different dosages in animal models. Studies have shown that lower doses of this compound effectively inhibit PRC2 activity without causing significant toxicity. Higher doses may lead to adverse effects, highlighting the importance of optimizing dosage for therapeutic applications. Threshold effects have been observed, with a clear dose-response relationship established in preclinical studies .
Metabolic Pathways
This compound is involved in metabolic pathways related to the inhibition of PRC2. The compound interacts with enzymes and cofactors associated with histone methylation, affecting metabolic flux and metabolite levels. The inhibition of PRC2 by this compound leads to changes in the epigenetic landscape, influencing various metabolic processes within the cell .
Transport and Distribution
Within cells and tissues, this compound is transported and distributed through interactions with specific transporters and binding proteins. These interactions influence the compound’s localization and accumulation, ensuring its effective inhibition of PRC2 activity. The transport and distribution of this compound are critical for its function as a chemical probe in biological studies .
Subcellular Localization
This compound’s subcellular localization is primarily within the nucleus, where it interacts with the PRC2 complex. The compound’s activity and function are influenced by its localization, with targeting signals and post-translational modifications directing it to specific compartments or organelles. This localization is essential for this compound’s role in inhibiting PRC2 and affecting gene expression .
Preparation Methods
Synthetic Routes and Reaction Conditions: Cloprostenol can be synthesized through a chemoenzymatic approach. The process involves the use of a dichloro-containing bicyclic ketone as a starting material. Key steps include a Baeyer-Villiger monooxygenase-catalyzed stereoselective oxidation and a ketoreductase-catalyzed diastereoselective reduction . The overall synthesis involves 11-12 steps with yields ranging from 3.8% to 8.4% .
Industrial Production Methods: Industrial production of cloprostenol sodium involves preparative liquid chromatography for separation and purification. This method ensures high product purity and is suitable for large-scale production . The process includes dissolving synthesized cloprostenol sodium in alcohol, followed by filtration and chromatographic separation .
Chemical Reactions Analysis
Types of Reactions: Cloprostenol undergoes various chemical reactions, including:
Oxidation: Stereoselective oxidation using Baeyer-Villiger monooxygenase.
Reduction: Diastereoselective reduction of enones using ketoreductase.
Substitution: Regioselective p-phenylbenzoylation of secondary alcohols.
Common Reagents and Conditions:
Oxidation: Baeyer-Villiger monooxygenase under mild conditions.
Reduction: Ketoreductase with high diastereoselectivity.
Substitution: Copper(II)-catalyzed reactions.
Major Products: The major products formed from these reactions include stereochemically defined intermediates essential for the synthesis of cloprostenol and related prostaglandins .
Scientific Research Applications
Cloprostenol has a wide range of applications in scientific research:
Chemistry: Used as a model compound for studying prostaglandin synthesis and reactions.
Biology: Investigated for its effects on luteal cells and progesterone production in various animal species.
Medicine: Employed in veterinary medicine to control reproductive cycles and induce parturition.
Industry: Utilized in the production of veterinary drugs and reproductive management tools.
Comparison with Similar Compounds
Cloprostenol is compared with other prostaglandin analogues such as:
- Bimatoprost
- Fluprostenol
- Travoprost
- Prostaglandin F2α
Uniqueness: Cloprostenol is unique due to its high luteolytic activity and specific applications in veterinary medicine . Its synthetic route and stereoselective synthesis also distinguish it from other prostaglandins .
Properties
IUPAC Name |
(3R,4S)-1-(7-fluoro-2,3-dihydro-1H-inden-1-yl)-N,N-dimethyl-4-[4-(4-methylsulfonylpiperazin-1-yl)phenyl]pyrrolidin-3-amine | |
---|---|---|
Source | PubChem | |
URL | https://pubchem.ncbi.nlm.nih.gov | |
Description | Data deposited in or computed by PubChem | |
InChI |
InChI=1S/C26H35FN4O2S/c1-28(2)25-18-30(24-12-9-20-5-4-6-23(27)26(20)24)17-22(25)19-7-10-21(11-8-19)29-13-15-31(16-14-29)34(3,32)33/h4-8,10-11,22,24-25H,9,12-18H2,1-3H3/t22-,24?,25+/m1/s1 | |
Source | PubChem | |
URL | https://pubchem.ncbi.nlm.nih.gov | |
Description | Data deposited in or computed by PubChem | |
InChI Key |
REVJNSVNICWODC-KIDMSAQOSA-N | |
Source | PubChem | |
URL | https://pubchem.ncbi.nlm.nih.gov | |
Description | Data deposited in or computed by PubChem | |
Canonical SMILES |
CN(C)C1CN(CC1C2=CC=C(C=C2)N3CCN(CC3)S(=O)(=O)C)C4CCC5=C4C(=CC=C5)F | |
Source | PubChem | |
URL | https://pubchem.ncbi.nlm.nih.gov | |
Description | Data deposited in or computed by PubChem | |
Isomeric SMILES |
CN(C)[C@H]1CN(C[C@@H]1C2=CC=C(C=C2)N3CCN(CC3)S(=O)(=O)C)C4CCC5=C4C(=CC=C5)F | |
Source | PubChem | |
URL | https://pubchem.ncbi.nlm.nih.gov | |
Description | Data deposited in or computed by PubChem | |
Molecular Formula |
C26H35FN4O2S | |
Source | PubChem | |
URL | https://pubchem.ncbi.nlm.nih.gov | |
Description | Data deposited in or computed by PubChem | |
Molecular Weight |
486.6 g/mol | |
Source | PubChem | |
URL | https://pubchem.ncbi.nlm.nih.gov | |
Description | Data deposited in or computed by PubChem | |
Q1: What is EAAT1, and why is it important?
A1: EAAT1 is a human excitatory amino acid transporter, a protein responsible for removing glutamate, a neurotransmitter, from the spaces between nerve cells. This process is crucial for proper brain function, as excessive glutamate can lead to neuronal damage. []
Q2: How do researchers study the structural changes of EAAT1 during glutamate transport?
A2: One method involves introducing cysteine pairs at specific locations within the EAAT1 protein. These cysteines can form disulfide bonds, acting as molecular tethers. By observing the formation and disruption of these bonds under different conditions, researchers can infer conformational changes associated with glutamate binding and transport. []
Q3: What did the research on EAAT1 reveal about the role of specific amino acid residues?
A3: The study highlighted the importance of Alanine 395 (Ala-395) in transmembrane domain 7 (TM7) of EAAT1. It was found to be spatially close to residues at the tips of two re-entrant loops, HP1 and HP2, suggesting their involvement in forming the substrate translocation pathway. []
Q4: How does the binding of glutamate or inhibitors affect the structural dynamics of EAAT1?
A4: The research showed that binding of glutamate or its inhibitors, like dl-threo-β-benzyloxyaspartate (dl-TBOA), prevents the formation of disulfide bonds between introduced cysteine pairs, indicating a shift in the relative positions of these residues. Conversely, sodium binding seems to facilitate crosslinking, highlighting the interplay of different factors in the transport cycle. []
Q5: What is the significance of genetic polymorphisms in disease susceptibility?
A5: Genetic polymorphisms, variations in our DNA sequence, can influence an individual's susceptibility to various diseases. These variations can affect how our bodies process toxins, repair DNA damage, and respond to environmental factors. [, ]
Q6: How are polymorphisms in DNA repair genes linked to lung cancer risk?
A6: Research suggests that specific polymorphisms in genes like XPD and APEX1, involved in DNA repair, can increase the risk of lung cancer development, especially in individuals exposed to carcinogens like those found in coal mines. These variations may hinder the body's ability to repair DNA damage caused by these substances, increasing the chance of mutations that lead to cancer. []
Q7: Can genetic polymorphisms be protective against certain diseases?
A7: Yes, some polymorphisms can have protective effects. For example, the study found that a large deletion in the GSTM1 gene, another detoxification enzyme gene, was associated with a decreased risk of lung cancer in coal mine workers. This suggests that individuals with this deletion might have a more efficient detoxification system, reducing their cancer risk. []
Q8: How can understanding genetic polymorphisms impact disease management?
A8: Identifying individuals with polymorphisms linked to increased disease susceptibility allows for personalized preventive measures, such as lifestyle modifications or increased surveillance. This knowledge can also guide the development of targeted therapies and interventions tailored to an individual's genetic makeup. [, , ]
Disclaimer and Information on In-Vitro Research Products
Please be aware that all articles and product information presented on BenchChem are intended solely for informational purposes. The products available for purchase on BenchChem are specifically designed for in-vitro studies, which are conducted outside of living organisms. In-vitro studies, derived from the Latin term "in glass," involve experiments performed in controlled laboratory settings using cells or tissues. It is important to note that these products are not categorized as medicines or drugs, and they have not received approval from the FDA for the prevention, treatment, or cure of any medical condition, ailment, or disease. We must emphasize that any form of bodily introduction of these products into humans or animals is strictly prohibited by law. It is essential to adhere to these guidelines to ensure compliance with legal and ethical standards in research and experimentation.