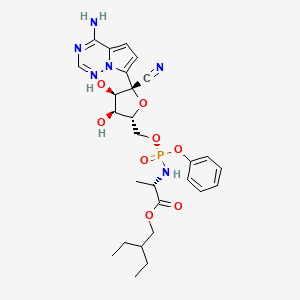
Remdesivir
Overview
Description
Remdesivir is an antiviral drug that is active against SARS-CoV-2, the cause of coronavirus disease 2019 (COVID-19) . It is administered by intravenous infusion in patients who are hospitalized or at high risk of severe COVID-19 . This compound acts by halting viral RNA production .
Synthesis Analysis
This compound is a nucleotide prodrug that exhibits broad-spectrum antiviral activities against RNA viruses . It has attracted considerable attention across the world for its effectiveness as an antiviral drug .Molecular Structure Analysis
This compound is a complex structure that inhibits RdRp and provides insight into the mechanisms of viral RNA replication . It is covalently inserted into the primer strand, leading to chain elongation termination .Chemical Reactions Analysis
This compound is an adenosine nucleotide analog prodrug that is metabolically activated to a nucleoside triphosphate metabolite . The active nucleoside triphosphate metabolite is incorporated into the SARS-CoV-2 RNA viral chains, preventing its replication .Physical And Chemical Properties Analysis
This compound is a low-molecular-weight (602.6 g/mol) prodrug with the molecular formula C27H35N6O8P . It undergoes metabolic activation to form the intracellular active triphosphate metabolite, GS-443902 .Scientific Research Applications
COVID-19 Treatment in Patients with Myasthenia Gravis : A study compared the efficacy of Dexamethasone and Remdesivir in treating COVID-19 patients who also have myasthenia gravis. It found that this compound, being an antiviral drug, targets the virus directly and is necessary for overall patient recovery when combined with other drugs like Dexamethasone (Bharadwaj et al., 2022).
Compassionate Use in Severe COVID-19 Cases : this compound was provided on a compassionate-use basis to patients with severe COVID-19. The study observed clinical improvement in 68% of the patients treated with this compound, suggesting its effectiveness in managing severe cases of COVID-19 (Grein et al., 2020).
Discovery and Development for COVID-19 Treatment : Another study provides an overview of this compound’s discovery and its mechanism of action. Originally evaluated for the Ebola outbreak, its effectiveness against SARS-CoV-2 was later identified, leading to its application in COVID-19 treatment (Eastman et al., 2020).
Mechanism of Action Against SARS-CoV-2 : Research on the mechanism of action of this compound shows that it inhibits the RNA-dependent RNA polymerase (RdRp) of coronaviruses, including SARS-CoV-2, stalling the replication process (Kokic et al., 2021).
Dosage Duration for Severe COVID-19 : A study comparing 5-day and 10-day dosing of this compound in severe COVID-19 patients found no significant difference in outcomes between the two durations, suggesting flexibility in treatment length (Goldman et al., 2020).
Randomized, Controlled Trial in Severe COVID-19 : A trial in China did not find a significant benefit of this compound compared to a control group in severe COVID-19 cases, indicating the need for further research to understand its efficacy (Wang et al., 2020).
Review of Clinical Data for COVID-19 Treatment : An analysis of clinical data up to May 2020 reviewed the efficacy and safety of this compound for COVID-19 patients, helping clinicians determine optimal patient populations for its use (Davis et al., 2020).
Review of this compound as a Promising Antiviral Candidate : A comprehensive review of this compound, including its mechanism, preclinical research, and clinical trials, discusses its potential as an effective treatment for SARS-CoV-2 infection (Liang et al., 2020).
Review of Antivirals this compound (GS-5734) and GS-441524 : This review synthesizes knowledge about this compound as a therapeutic option for coronaviruses, focusing on information relevant to public health practitioners (Amirian & Levy, 2020).
Update on this compound for Adults With COVID-19 : This update from a living rapid review includes information from newly published large multinational randomized controlled trials, offering insights into the effectiveness of this compound for COVID-19 treatment (Kaka et al., 2021).
Mechanism of Action
Target of Action
Remdesivir, also known as GS-5734, is a nucleoside analog . Its primary target is the RNA-dependent RNA polymerase (RdRp) enzyme complex of RNA viruses . This enzyme complex is crucial for the replication of the viral genome .
Mode of Action
This compound acts by inhibiting the replication of the virus . It is metabolically activated to form an intracellular active triphosphate metabolite, GS-443902 . This active metabolite is incorporated into the viral RNA chains, thereby inhibiting the RdRp and preventing the virus from growing and spreading in the body . It does this by terminating RNA transcription prematurely .
Biochemical Pathways
This compound affects the biochemical pathway of viral replication. By inhibiting the RdRp enzyme complex, it disrupts the replication of the viral RNA genome . This results in the termination of the viral RNA chains, thereby preventing the virus from replicating and spreading within the host organism .
Pharmacokinetics
This compound exhibits linear pharmacokinetics following single-dose intravenous administration . It undergoes metabolic activation to form the intracellular active triphosphate metabolite, GS-443902 . The major metabolite GS-441524 accumulates approximately 1.9-fold in plasma following multiple doses of this compound 150 mg once daily for 7 or 14 days . The clinical dose regimen of a 200-mg loading dose on day 1 followed by 100-mg maintenance doses for 4 or 9 days was selected for further evaluation of pharmacokinetics and safety .
Result of Action
The result of this compound’s action is the inhibition of viral replication. This can help the body’s immune system effectively control the infection by stopping the virus from growing and spreading in the body . It has demonstrated in vitro and in vivo activity against SARS-CoV-2 .
Action Environment
The efficacy of this compound can be influenced by various environmental factors. For instance, the drug’s effectiveness may vary depending on the patient’s health status and the presence of other diseases . Additionally, the drug’s pharmacokinetics can be affected by factors such as the patient’s age, weight, and renal function .
Safety and Hazards
Future Directions
properties
IUPAC Name |
2-ethylbutyl (2S)-2-[[[(2R,3S,4R,5R)-5-(4-aminopyrrolo[2,1-f][1,2,4]triazin-7-yl)-5-cyano-3,4-dihydroxyoxolan-2-yl]methoxy-phenoxyphosphoryl]amino]propanoate | |
---|---|---|
Source | PubChem | |
URL | https://pubchem.ncbi.nlm.nih.gov | |
Description | Data deposited in or computed by PubChem | |
InChI |
InChI=1S/C27H35N6O8P/c1-4-18(5-2)13-38-26(36)17(3)32-42(37,41-19-9-7-6-8-10-19)39-14-21-23(34)24(35)27(15-28,40-21)22-12-11-20-25(29)30-16-31-33(20)22/h6-12,16-18,21,23-24,34-35H,4-5,13-14H2,1-3H3,(H,32,37)(H2,29,30,31)/t17-,21+,23+,24+,27-,42-/m0/s1 | |
Source | PubChem | |
URL | https://pubchem.ncbi.nlm.nih.gov | |
Description | Data deposited in or computed by PubChem | |
InChI Key |
RWWYLEGWBNMMLJ-YSOARWBDSA-N | |
Source | PubChem | |
URL | https://pubchem.ncbi.nlm.nih.gov | |
Description | Data deposited in or computed by PubChem | |
Canonical SMILES |
CCC(CC)COC(=O)C(C)NP(=O)(OCC1C(C(C(O1)(C#N)C2=CC=C3N2N=CN=C3N)O)O)OC4=CC=CC=C4 | |
Source | PubChem | |
URL | https://pubchem.ncbi.nlm.nih.gov | |
Description | Data deposited in or computed by PubChem | |
Isomeric SMILES |
CCC(CC)COC(=O)[C@H](C)N[P@](=O)(OC[C@@H]1[C@H]([C@H]([C@](O1)(C#N)C2=CC=C3N2N=CN=C3N)O)O)OC4=CC=CC=C4 | |
Source | PubChem | |
URL | https://pubchem.ncbi.nlm.nih.gov | |
Description | Data deposited in or computed by PubChem | |
Molecular Formula |
C27H35N6O8P | |
Source | PubChem | |
URL | https://pubchem.ncbi.nlm.nih.gov | |
Description | Data deposited in or computed by PubChem | |
DSSTOX Substance ID |
DTXSID701022537 | |
Record name | Remdesivir | |
Source | EPA DSSTox | |
URL | https://comptox.epa.gov/dashboard/DTXSID701022537 | |
Description | DSSTox provides a high quality public chemistry resource for supporting improved predictive toxicology. | |
Molecular Weight |
602.6 g/mol | |
Source | PubChem | |
URL | https://pubchem.ncbi.nlm.nih.gov | |
Description | Data deposited in or computed by PubChem | |
Mechanism of Action |
COVID-19 is caused by the positive-sense RNA virus severe acute respiratory syndrome coronavirus 2 (SARS-CoV-2). Replication of the viral genome is a key step in the infectious cycle of RNA viruses, including those of the _Filoviridae_, _Paramyxoviridae_, _Pneumoviridae_, and _Coronaviridae_ families, and is carried out by viral RNA-dependent RNA polymerase (RdRp) enzymes or enzyme complexes. For both SARS-CoV and SARS-CoV-2, the RdRp comprises nsp7, nsp8, and nsp12 subunits under physiological conditions, although functional RdRp complexes can be reassembled _in vitro_ that incorporate only the nsp8 and nsp12 subunits, similar to the Middle East respiratory syndrome coronavirus (MERS-CoV). Remdesivir is a phosphoramidite prodrug of a 1'-cyano-substituted adenosine nucleotide analogue that competes with ATP for incorporation into newly synthesized viral RNA by the corresponding RdRp complex. Remdesivir enters cells before being cleaved to its monophosphate form through the action of either carboxylesterase 1 or cathepsin A; it is subsequently phosphorylated by undescribed kinases to yield its active triphosphate form remdesivir triphosphate (RDV-TP or GS-443902). RDV-TP is efficiently incorporated by the SARS-CoV-2 RdRp complex, with a 3.65-fold selectivity for RDV-TP over endogenous ATP. Unlike some nucleoside analogues, remdesivir provides a free 3'-hydroxyl group that allows for continued chain elongation. However, modelling and _in vitro_ experiments suggest that at _i_ + 4 (corresponding to the position for the incorporation of the fourth nucleotide following RDV-TP incorporation), the 1'-cyano group of remdesivir sterically clashes with Ser-861 of the RdRp, preventing further enzyme translocation and terminating replication at position _i_ + 3. This mechanism was essentially identical between SARS-CoV, SARS-CoV-2, and MERS-CoV, and genomic comparisons reveal that Ser-861 is conserved across alpha-, beta-, and deltacoronaviruses, suggesting remdesivir may possess broad antiviral activity. Considerations for the use of nucleotide analogues like remdesivir include the possible accumulation of resistance mutations. Excision of analogues through the 3'-5' exonuclease (ExoN) activity of replication complexes, mediated in SARS-CoV by the nsp14 subunit, is of possible concern. Murine hepatitis viruses (MHVs) engineered to lack ExoN activity are approximately 4-fold more susceptible to remdesivir, supporting the proposed mechanism of action. However, the relatively mild benefit of ExoN activity to remdesivir resistance is proposed to involve its delayed chain termination mechanism, whereby additional endogenous nucleotides are incorporated following RDV-TP. In addition, serial passage of MHV in increasing concentrations of the remdesivir parent molecule [GS-441524] led to the development of resistance mutations F476L and V553L, which maintain activity when transferred to SARS-CoV. However, these mutant viruses are less fit than wild-type in both competition assays and _in vivo_ in the absence of selective pressure. To date, no clinical data on SARS-CoV-2 resistance to remdesivir have been described. | |
Record name | Remdesivir | |
Source | DrugBank | |
URL | https://www.drugbank.ca/drugs/DB14761 | |
Description | The DrugBank database is a unique bioinformatics and cheminformatics resource that combines detailed drug (i.e. chemical, pharmacological and pharmaceutical) data with comprehensive drug target (i.e. sequence, structure, and pathway) information. | |
Explanation | Creative Common's Attribution-NonCommercial 4.0 International License (http://creativecommons.org/licenses/by-nc/4.0/legalcode) | |
CAS RN |
1809249-37-3 | |
Record name | L-Alanine, N-[(S)-hydroxyphenoxyphosphinyl]-, 2-ethylbutyl ester, 6-ester with 2-C-(4-aminopyrrolo[2,1-f][1,2,4]triazin-7-yl)-2,5-anhydro-D-altrononitrile | |
Source | CAS Common Chemistry | |
URL | https://commonchemistry.cas.org/detail?cas_rn=1809249-37-3 | |
Description | CAS Common Chemistry is an open community resource for accessing chemical information. Nearly 500,000 chemical substances from CAS REGISTRY cover areas of community interest, including common and frequently regulated chemicals, and those relevant to high school and undergraduate chemistry classes. This chemical information, curated by our expert scientists, is provided in alignment with our mission as a division of the American Chemical Society. | |
Explanation | The data from CAS Common Chemistry is provided under a CC-BY-NC 4.0 license, unless otherwise stated. | |
Record name | Remdesivir [USAN] | |
Source | ChemIDplus | |
URL | https://pubchem.ncbi.nlm.nih.gov/substance/?source=chemidplus&sourceid=1809249373 | |
Description | ChemIDplus is a free, web search system that provides access to the structure and nomenclature authority files used for the identification of chemical substances cited in National Library of Medicine (NLM) databases, including the TOXNET system. | |
Record name | Remdesivir | |
Source | DrugBank | |
URL | https://www.drugbank.ca/drugs/DB14761 | |
Description | The DrugBank database is a unique bioinformatics and cheminformatics resource that combines detailed drug (i.e. chemical, pharmacological and pharmaceutical) data with comprehensive drug target (i.e. sequence, structure, and pathway) information. | |
Explanation | Creative Common's Attribution-NonCommercial 4.0 International License (http://creativecommons.org/licenses/by-nc/4.0/legalcode) | |
Record name | Remdesivir | |
Source | EPA DSSTox | |
URL | https://comptox.epa.gov/dashboard/DTXSID701022537 | |
Description | DSSTox provides a high quality public chemistry resource for supporting improved predictive toxicology. | |
Record name | 2-ethylbutyl (2S)-2-[[[(2R,3S,4R,5R)-5-(4-aminopyrrolo[2,1-f][1,2,4]triazin-7-yl)-5-cyano-3,4-dihydroxyoxolan-2-yl]methoxy-phenoxyphosphoryl]amino]propanoate | |
Source | European Chemicals Agency (ECHA) | |
URL | https://echa.europa.eu/information-on-chemicals | |
Description | The European Chemicals Agency (ECHA) is an agency of the European Union which is the driving force among regulatory authorities in implementing the EU's groundbreaking chemicals legislation for the benefit of human health and the environment as well as for innovation and competitiveness. | |
Explanation | Use of the information, documents and data from the ECHA website is subject to the terms and conditions of this Legal Notice, and subject to other binding limitations provided for under applicable law, the information, documents and data made available on the ECHA website may be reproduced, distributed and/or used, totally or in part, for non-commercial purposes provided that ECHA is acknowledged as the source: "Source: European Chemicals Agency, http://echa.europa.eu/". Such acknowledgement must be included in each copy of the material. ECHA permits and encourages organisations and individuals to create links to the ECHA website under the following cumulative conditions: Links can only be made to webpages that provide a link to the Legal Notice page. | |
Record name | REMDESIVIR | |
Source | FDA Global Substance Registration System (GSRS) | |
URL | https://gsrs.ncats.nih.gov/ginas/app/beta/substances/3QKI37EEHE | |
Description | The FDA Global Substance Registration System (GSRS) enables the efficient and accurate exchange of information on what substances are in regulated products. Instead of relying on names, which vary across regulatory domains, countries, and regions, the GSRS knowledge base makes it possible for substances to be defined by standardized, scientific descriptions. | |
Explanation | Unless otherwise noted, the contents of the FDA website (www.fda.gov), both text and graphics, are not copyrighted. They are in the public domain and may be republished, reprinted and otherwise used freely by anyone without the need to obtain permission from FDA. Credit to the U.S. Food and Drug Administration as the source is appreciated but not required. | |
Disclaimer and Information on In-Vitro Research Products
Please be aware that all articles and product information presented on BenchChem are intended solely for informational purposes. The products available for purchase on BenchChem are specifically designed for in-vitro studies, which are conducted outside of living organisms. In-vitro studies, derived from the Latin term "in glass," involve experiments performed in controlled laboratory settings using cells or tissues. It is important to note that these products are not categorized as medicines or drugs, and they have not received approval from the FDA for the prevention, treatment, or cure of any medical condition, ailment, or disease. We must emphasize that any form of bodily introduction of these products into humans or animals is strictly prohibited by law. It is essential to adhere to these guidelines to ensure compliance with legal and ethical standards in research and experimentation.