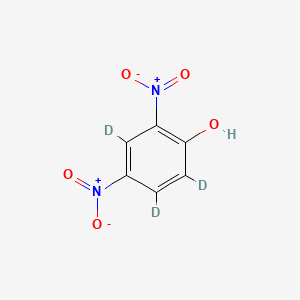
2,4-Dinitrophenol-d3
Overview
Description
2,4-Dinitrophenol-d3: is a deuterated derivative of 2,4-dinitrophenol, where three hydrogen atoms are replaced by deuterium. This compound is primarily used in scientific research, particularly in nuclear magnetic resonance (NMR) and mass spectrometry as an internal standard . It shares similar chemical properties with 2,4-dinitrophenol, which is known for its role as a metabolic uncoupler and its historical use in weight loss treatments .
Mechanism of Action
Target of Action
The primary target of 2,4-Dinitrophenol-d3 is the mitochondrial membrane . More specifically, it acts as a protonophore, an agent that can shuttle protons (hydrogen cations) across biological membranes . It disrupts the proton gradient across the mitochondrial membrane, which is crucial for the cell’s production of most of its ATP chemical energy .
Mode of Action
This compound interacts with its target, the mitochondrial membrane, by dissipating the proton gradient across it . This action collapses the proton motive force that the cell uses to produce most of its ATP chemical energy . Instead of producing ATP, the energy of the proton gradient is lost as heat .
Biochemical Pathways
The primary biochemical pathway affected by this compound is oxidative phosphorylation . By uncoupling oxidative phosphorylation, this compound prevents the efficient production of ATP, leading to a rapid loss of ATP as heat . This action can significantly affect cellular metabolism and energy production .
Pharmacokinetics
Information about the pharmacokinetics of this compound in humans is limited . It is known that this compound exhibits significant nonlinear pharmacokinetics, attributed to nonlinear plasma protein binding and nonlinear partitioning into liver and kidney . After administration, distribution of this compound to tissues is limited . The compound is metabolized via nitro reduction .
Result of Action
The action of this compound results in a rapid loss of ATP as heat, leading to uncontrolled hyperthermia . This can cause a general disturbance of cell metabolism, resulting in a need for cells to consume excessive amounts of oxygen to synthesize the essential adenine nucleotide required for cell survival .
Action Environment
The action, efficacy, and stability of this compound can be influenced by various environmental factors. It’s important to note that the compound’s action can be dangerous, leading to severe toxicities, and it has been banned for human use in many countries due to its risk of death and toxic side effects .
Biochemical Analysis
Biochemical Properties
2,4-Dinitrophenol-d3 plays a significant role in biochemical reactions. It acts as a proton carrier, transporting protons across the mitochondrial membrane . This action disrupts the normal proton gradient essential for ATP synthesis, leading to a rapid loss of ATP and an increase in metabolic rate . The compound interacts with various enzymes and proteins, including adenine nucleotide translocase (ANT) and mitochondrial uncoupling proteins (UCP1-UCP3), enhancing the protonophoric action of this compound .
Cellular Effects
The effects of this compound on cells are profound. It influences cell function by disrupting ATP production, which can affect various cellular processes, including cell signaling pathways, gene expression, and cellular metabolism . The compound causes dose-dependent mitochondrial uncoupling, leading to uncontrolled hyperthermia in case of overdose .
Molecular Mechanism
The molecular mechanism of this compound involves its ability to carry and release protons as it freely diffuses through the mitochondrial membrane . This action uncouples oxidative phosphorylation, causing cells to catabolize more stores of carbohydrates and fats . The compound also binds to ANT1, with arginine 79 of ANT1 being crucial for the DNP-mediated increase of membrane conductance .
Temporal Effects in Laboratory Settings
Over time, the effects of this compound can change. In laboratory settings, it has been observed that the compound shows attenuation impact on oxidative stress depending on both dose and time . Information on the product’s stability, degradation, and any long-term effects on cellular function observed in in vitro or in vivo studies is still being researched .
Dosage Effects in Animal Models
In animal models, the effects of this compound vary with different dosages. Studies have shown that the compound delays disease onset and improves motor coordination and muscle contractile function in hSOD1G93A mice, a model for Amyotrophic Lateral Sclerosis, when administered at doses of 0.5 or 1.0 mg/kg .
Metabolic Pathways
This compound is involved in the metabolic pathway of oxidative phosphorylation. It disrupts this pathway by carrying protons across the mitochondrial membrane, thereby uncoupling the process and leading to a rapid loss of ATP .
Transport and Distribution
This compound is transported across the mitochondrial membrane where it carries and releases protons
Subcellular Localization
The subcellular localization of this compound is primarily in the mitochondria, where it carries and releases protons across the mitochondrial membrane . This action disrupts the normal proton gradient, leading to a rapid loss of ATP and an increase in metabolic rate .
Preparation Methods
Synthetic Routes and Reaction Conditions:
The preparation of 2,4-dinitrophenol-d3 typically involves the nitration of phenol-d3. The process can be summarized as follows:
Nitration of Phenol-d3: Phenol-d3 is treated with a mixture of concentrated sulfuric acid and nitric acid at a controlled temperature to introduce nitro groups at the 2 and 4 positions of the aromatic ring.
Purification: The crude product is purified through recrystallization, often using solvents like ethanol or acetone to obtain pure this compound.
Industrial Production Methods:
Industrial production of this compound follows similar steps but on a larger scale. The process involves:
Hydrolysis: Adding water to a hydrolysis kettle and heating to 60°C.
Addition of 2,4-Dinitrochlorobenzene: Molten 2,4-dinitrochlorobenzene is added and heated to 90°C.
Sodium Hydroxide Solution: Gradually adding a 30% sodium hydroxide solution while maintaining the temperature at 102-104°C.
Crystallization: Cooling the mixture, filtering the precipitated sodium salt, dissolving it in water, and acidifying to precipitate this compound crystals.
Chemical Reactions Analysis
Types of Reactions:
2,4-Dinitrophenol-d3 undergoes various chemical reactions, including:
Reduction: It can be reduced to 2,4-diaminophenol-d3 using reducing agents like sodium dithionite.
Substitution: The nitro groups can be substituted with other functional groups under specific conditions.
Oxidation: It can be oxidized to form quinones under strong oxidizing conditions.
Common Reagents and Conditions:
Reduction: Sodium dithionite in an aqueous medium.
Substitution: Nucleophiles like amines or thiols in the presence of a base.
Oxidation: Strong oxidizing agents like potassium permanganate or chromium trioxide.
Major Products:
Reduction: 2,4-Diaminophenol-d3.
Substitution: Various substituted phenols depending on the nucleophile used.
Oxidation: Quinones and other oxidized derivatives.
Scientific Research Applications
2,4-Dinitrophenol-d3 is widely used in scientific research due to its unique properties:
Nuclear Magnetic Resonance (NMR): As an internal standard for calibrating chemical shifts.
Mass Spectrometry: As a reference compound for accurate mass determination.
Biochemical Studies: Investigating metabolic pathways and enzyme activities.
Pharmacokinetics: Studying the distribution and metabolism of drugs
Comparison with Similar Compounds
2,4-Dinitrophenol: The non-deuterated version, known for its metabolic uncoupling properties.
2,6-Dinitrophenol: Another isomer with similar properties but different reactivity.
4-Nitrophenol: A mono-nitrated phenol with different chemical behavior
Uniqueness:
2,4-Dinitrophenol-d3 is unique due to the presence of deuterium atoms, which makes it particularly useful in NMR and mass spectrometry for precise measurements and as an internal standard .
Properties
IUPAC Name |
2,3,5-trideuterio-4,6-dinitrophenol | |
---|---|---|
Source | PubChem | |
URL | https://pubchem.ncbi.nlm.nih.gov | |
Description | Data deposited in or computed by PubChem | |
InChI |
InChI=1S/C6H4N2O5/c9-6-2-1-4(7(10)11)3-5(6)8(12)13/h1-3,9H/i1D,2D,3D | |
Source | PubChem | |
URL | https://pubchem.ncbi.nlm.nih.gov | |
Description | Data deposited in or computed by PubChem | |
InChI Key |
UFBJCMHMOXMLKC-CBYSEHNBSA-N | |
Source | PubChem | |
URL | https://pubchem.ncbi.nlm.nih.gov | |
Description | Data deposited in or computed by PubChem | |
Canonical SMILES |
C1=CC(=C(C=C1[N+](=O)[O-])[N+](=O)[O-])O | |
Source | PubChem | |
URL | https://pubchem.ncbi.nlm.nih.gov | |
Description | Data deposited in or computed by PubChem | |
Isomeric SMILES |
[2H]C1=C(C(=C(C(=C1[N+](=O)[O-])[2H])[N+](=O)[O-])O)[2H] | |
Source | PubChem | |
URL | https://pubchem.ncbi.nlm.nih.gov | |
Description | Data deposited in or computed by PubChem | |
Molecular Formula |
C6H4N2O5 | |
Source | PubChem | |
URL | https://pubchem.ncbi.nlm.nih.gov | |
Description | Data deposited in or computed by PubChem | |
DSSTOX Substance ID |
DTXSID10745652 | |
Record name | 2,4-Dinitro(~2~H_3_)phenol | |
Source | EPA DSSTox | |
URL | https://comptox.epa.gov/dashboard/DTXSID10745652 | |
Description | DSSTox provides a high quality public chemistry resource for supporting improved predictive toxicology. | |
Molecular Weight |
187.12 g/mol | |
Source | PubChem | |
URL | https://pubchem.ncbi.nlm.nih.gov | |
Description | Data deposited in or computed by PubChem | |
CAS No. |
93951-77-0 | |
Record name | 2,4-Dinitro(~2~H_3_)phenol | |
Source | EPA DSSTox | |
URL | https://comptox.epa.gov/dashboard/DTXSID10745652 | |
Description | DSSTox provides a high quality public chemistry resource for supporting improved predictive toxicology. | |
Record name | 93951-77-0 | |
Source | European Chemicals Agency (ECHA) | |
URL | https://echa.europa.eu/information-on-chemicals | |
Description | The European Chemicals Agency (ECHA) is an agency of the European Union which is the driving force among regulatory authorities in implementing the EU's groundbreaking chemicals legislation for the benefit of human health and the environment as well as for innovation and competitiveness. | |
Explanation | Use of the information, documents and data from the ECHA website is subject to the terms and conditions of this Legal Notice, and subject to other binding limitations provided for under applicable law, the information, documents and data made available on the ECHA website may be reproduced, distributed and/or used, totally or in part, for non-commercial purposes provided that ECHA is acknowledged as the source: "Source: European Chemicals Agency, http://echa.europa.eu/". Such acknowledgement must be included in each copy of the material. ECHA permits and encourages organisations and individuals to create links to the ECHA website under the following cumulative conditions: Links can only be made to webpages that provide a link to the Legal Notice page. | |
Synthesis routes and methods I
Procedure details
Synthesis routes and methods II
Procedure details
Disclaimer and Information on In-Vitro Research Products
Please be aware that all articles and product information presented on BenchChem are intended solely for informational purposes. The products available for purchase on BenchChem are specifically designed for in-vitro studies, which are conducted outside of living organisms. In-vitro studies, derived from the Latin term "in glass," involve experiments performed in controlled laboratory settings using cells or tissues. It is important to note that these products are not categorized as medicines or drugs, and they have not received approval from the FDA for the prevention, treatment, or cure of any medical condition, ailment, or disease. We must emphasize that any form of bodily introduction of these products into humans or animals is strictly prohibited by law. It is essential to adhere to these guidelines to ensure compliance with legal and ethical standards in research and experimentation.