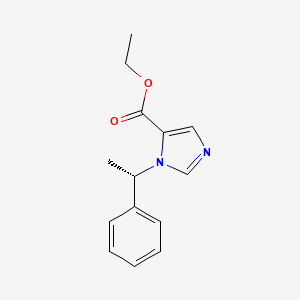
S-(-)-Etomidate
Overview
Description
S-ETOMIDATE is a stereoisomer of etomidate, a hypnotic agent used for the induction of anesthesia. It is an imidazole-based compound that acts as a positive allosteric modulator on the γ-aminobutyric acid type A receptor, enhancing the effect of the inhibitory neurotransmitter γ-aminobutyric acid . S-ETOMIDATE is known for its stable cardiorespiratory profile and minimal respiratory depression .
Preparation Methods
Synthetic Routes and Reaction Conditions
The synthesis of S-ETOMIDATE involves the esterification of an imidazole derivative. The process typically includes the following steps:
Formation of the imidazole ring: This is achieved through the cyclization of appropriate precursors under acidic or basic conditions.
Esterification: The imidazole derivative is then esterified with an alcohol, such as ethanol, in the presence of a catalyst like sulfuric acid.
Purification: The final product is purified using techniques such as recrystallization or chromatography to obtain the desired stereoisomer.
Industrial Production Methods
Industrial production of S-ETOMIDATE follows similar synthetic routes but on a larger scale. The process is optimized for yield and purity, often involving continuous flow reactors and automated purification systems to ensure consistency and efficiency .
Chemical Reactions Analysis
Types of Reactions
S-ETOMIDATE undergoes several types of chemical reactions, including:
Hydrolysis: In the presence of water and esterases, S-ETOMIDATE is hydrolyzed to its carboxylic acid form.
Oxidation: It can be oxidized under specific conditions to form various oxidized derivatives.
Substitution: The imidazole ring can undergo substitution reactions with electrophiles or nucleophiles.
Common Reagents and Conditions
Hydrolysis: Esterases or acidic/basic conditions.
Oxidation: Oxidizing agents such as potassium permanganate or hydrogen peroxide.
Substitution: Electrophiles like alkyl halides or nucleophiles like amines.
Major Products Formed
Hydrolysis: Carboxylic acid derivative.
Oxidation: Oxidized imidazole derivatives.
Substitution: Substituted imidazole compounds.
Scientific Research Applications
S-ETOMIDATE has a wide range of scientific research applications:
Mechanism of Action
S-ETOMIDATE exerts its effects by binding to a distinct site on the γ-aminobutyric acid type A receptor, increasing the duration of chloride ion channel opening. This leads to hyperpolarization of neurons, inhibiting neuronal firing and resulting in sedation, hypnosis, and anesthesia . The compound’s action is mediated through the central nervous system, specifically targeting the reticular-activating system .
Comparison with Similar Compounds
Similar Compounds
Etomidate: The racemic mixture of which S-ETOMIDATE is a stereoisomer.
Propofol: Another intravenous anesthetic with a different mechanism of action.
Uniqueness
S-ETOMIDATE stands out due to its stable cardiorespiratory profile and minimal respiratory depression compared to other anesthetics like propofol and thiopental . Unlike etomidate, S-ETOMIDATE has a reduced impact on adrenocortical function, making it a safer option for prolonged use .
Properties
IUPAC Name |
ethyl 3-[(1S)-1-phenylethyl]imidazole-4-carboxylate | |
---|---|---|
Source | PubChem | |
URL | https://pubchem.ncbi.nlm.nih.gov | |
Description | Data deposited in or computed by PubChem | |
InChI |
InChI=1S/C14H16N2O2/c1-3-18-14(17)13-9-15-10-16(13)11(2)12-7-5-4-6-8-12/h4-11H,3H2,1-2H3/t11-/m0/s1 | |
Source | PubChem | |
URL | https://pubchem.ncbi.nlm.nih.gov | |
Description | Data deposited in or computed by PubChem | |
InChI Key |
NPUKDXXFDDZOKR-NSHDSACASA-N | |
Source | PubChem | |
URL | https://pubchem.ncbi.nlm.nih.gov | |
Description | Data deposited in or computed by PubChem | |
Canonical SMILES |
CCOC(=O)C1=CN=CN1C(C)C2=CC=CC=C2 | |
Source | PubChem | |
URL | https://pubchem.ncbi.nlm.nih.gov | |
Description | Data deposited in or computed by PubChem | |
Isomeric SMILES |
CCOC(=O)C1=CN=CN1[C@@H](C)C2=CC=CC=C2 | |
Source | PubChem | |
URL | https://pubchem.ncbi.nlm.nih.gov | |
Description | Data deposited in or computed by PubChem | |
Molecular Formula |
C14H16N2O2 | |
Source | PubChem | |
URL | https://pubchem.ncbi.nlm.nih.gov | |
Description | Data deposited in or computed by PubChem | |
Molecular Weight |
244.29 g/mol | |
Source | PubChem | |
URL | https://pubchem.ncbi.nlm.nih.gov | |
Description | Data deposited in or computed by PubChem | |
CAS No. |
56649-47-9 | |
Record name | 1H-Imidazole-5-carboxylic acid, 1-[(1S)-1-phenylethyl]-, ethyl ester | |
Source | European Chemicals Agency (ECHA) | |
URL | https://echa.europa.eu/information-on-chemicals | |
Description | The European Chemicals Agency (ECHA) is an agency of the European Union which is the driving force among regulatory authorities in implementing the EU's groundbreaking chemicals legislation for the benefit of human health and the environment as well as for innovation and competitiveness. | |
Explanation | Use of the information, documents and data from the ECHA website is subject to the terms and conditions of this Legal Notice, and subject to other binding limitations provided for under applicable law, the information, documents and data made available on the ECHA website may be reproduced, distributed and/or used, totally or in part, for non-commercial purposes provided that ECHA is acknowledged as the source: "Source: European Chemicals Agency, http://echa.europa.eu/". Such acknowledgement must be included in each copy of the material. ECHA permits and encourages organisations and individuals to create links to the ECHA website under the following cumulative conditions: Links can only be made to webpages that provide a link to the Legal Notice page. | |
Retrosynthesis Analysis
AI-Powered Synthesis Planning: Our tool employs the Template_relevance Pistachio, Template_relevance Bkms_metabolic, Template_relevance Pistachio_ringbreaker, Template_relevance Reaxys, Template_relevance Reaxys_biocatalysis model, leveraging a vast database of chemical reactions to predict feasible synthetic routes.
One-Step Synthesis Focus: Specifically designed for one-step synthesis, it provides concise and direct routes for your target compounds, streamlining the synthesis process.
Accurate Predictions: Utilizing the extensive PISTACHIO, BKMS_METABOLIC, PISTACHIO_RINGBREAKER, REAXYS, REAXYS_BIOCATALYSIS database, our tool offers high-accuracy predictions, reflecting the latest in chemical research and data.
Strategy Settings
Precursor scoring | Relevance Heuristic |
---|---|
Min. plausibility | 0.01 |
Model | Template_relevance |
Template Set | Pistachio/Bkms_metabolic/Pistachio_ringbreaker/Reaxys/Reaxys_biocatalysis |
Top-N result to add to graph | 6 |
Feasible Synthetic Routes
Q1: How does S-etomidate interact with its target and what are the downstream effects?
A: Similar to its enantiomer, S-etomidate acts as a positive allosteric modulator of GABAA receptors in the brain. [, ] These receptors are ligand-gated chloride channels responsible for mediating inhibitory neurotransmission in the central nervous system. By binding to a distinct site on the receptor, S-etomidate enhances the effects of the inhibitory neurotransmitter GABA, leading to increased chloride influx into neurons. This hyperpolarization reduces neuronal excitability, ultimately resulting in sedative, hypnotic, and amnesic effects. []
Q2: What is the significance of the lower adrenocortical suppression observed with S-etomidate?
A: One crucial finding regarding S-etomidate is its significantly reduced potency in suppressing adrenocortical function compared to R-etomidate. [] This difference is particularly important because the clinical use of R-etomidate is limited by its potential to induce adrenal insufficiency. The decreased adrenocortical suppression associated with S-etomidate suggests that it might possess a safer pharmacological profile in terms of endocrine effects. [] This finding has sparked interest in exploring S-etomidate or its analogs as potential alternatives to R-etomidate with reduced endocrine side effects.
Q3: What insights do metabolism studies provide about S-etomidate?
A: In vitro studies using rat liver fractions have shown that S-etomidate undergoes metabolism primarily through ester hydrolysis, similar to R-etomidate. [] This process leads to the formation of an inactive metabolite. [] Interestingly, the rate of metabolism was observed to be slower for S-etomidate compared to R-etomidate, suggesting potential differences in their pharmacokinetic profiles. [] Further in vivo studies in rats confirmed that ester hydrolysis is the primary metabolic pathway for both enantiomers, resulting in a single amphoteric metabolite that is primarily excreted in urine. []
Q4: How is the chiral purity of S-etomidate determined?
A: A robust capillary electrophoresis method has been developed and validated to assess the enantiomeric purity of etomidate, including the determination of S-etomidate. [] This method employs a dual cyclodextrin system as a chiral selector, allowing for the separation and quantification of both enantiomers. [] This technique is crucial for ensuring the quality control of S-etomidate used in research and potential therapeutic development.
Q5: What are the implications of the research on S-etomidate for future drug development?
A: The findings from various studies on S-etomidate contribute significantly to our understanding of the structure-activity relationships of etomidate analogs. The distinct pharmacological profile of S-etomidate, particularly its reduced adrenocortical suppression, highlights the potential of modifying the chiral center of etomidate to develop novel therapeutics with improved safety profiles. [] This knowledge serves as a foundation for designing and synthesizing new etomidate derivatives with enhanced therapeutic indices and reduced adverse effects for various clinical applications.
Disclaimer and Information on In-Vitro Research Products
Please be aware that all articles and product information presented on BenchChem are intended solely for informational purposes. The products available for purchase on BenchChem are specifically designed for in-vitro studies, which are conducted outside of living organisms. In-vitro studies, derived from the Latin term "in glass," involve experiments performed in controlled laboratory settings using cells or tissues. It is important to note that these products are not categorized as medicines or drugs, and they have not received approval from the FDA for the prevention, treatment, or cure of any medical condition, ailment, or disease. We must emphasize that any form of bodily introduction of these products into humans or animals is strictly prohibited by law. It is essential to adhere to these guidelines to ensure compliance with legal and ethical standards in research and experimentation.