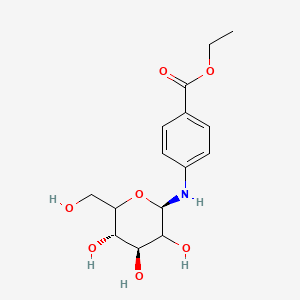
Benzocaine N-|A-D-Glucoside
Overview
Description
Benzocaine N-β-D-Glucoside (CAS No. 28315-50-6) is a glycosidic derivative of benzocaine (ethyl 4-aminobenzoate), a widely used ester-type local anesthetic. The compound is synthesized by conjugating benzocaine with a β-D-glucose moiety via an N-glycosidic bond, resulting in the molecular formula C₁₅H₂₁NO₇ and a molar mass of 327.33 g/mol . Key physicochemical properties include a melting point of 174°C, a flash point of 303.5°C, and a storage requirement of -20°C to maintain stability.
Preparation Methods
Synthetic Routes and Reaction Conditions: The synthesis of Benzocaine N-|A-D-Glucoside typically involves the glycosylation of benzocaine. One common method is the reaction of benzocaine with a glucosyl donor under acidic or basic conditions to form the glycosidic bond. The reaction can be catalyzed by acids such as hydrochloric acid or sulfuric acid, or by bases such as sodium hydroxide .
Industrial Production Methods: In industrial settings, the production of this compound may involve large-scale glycosylation reactions using optimized conditions to ensure high yield and purity. The process may include steps such as purification by recrystallization or chromatography to isolate the desired product .
Chemical Reactions Analysis
Types of Reactions: Benzocaine N-|A-D-Glucoside can undergo various chemical reactions, including:
Oxidation: The compound can be oxidized to form corresponding quinones.
Reduction: Reduction reactions can convert the compound back to its parent amine form.
Substitution: Nucleophilic substitution reactions can occur at the glucoside moiety.
Common Reagents and Conditions:
Oxidation: Reagents such as potassium permanganate or chromium trioxide.
Reduction: Reagents like sodium borohydride or lithium aluminum hydride.
Substitution: Nucleophiles such as sodium azide or thiols.
Major Products Formed:
Oxidation: Quinones and other oxidized derivatives.
Reduction: Parent amine compounds.
Substitution: Various substituted glucosides.
Scientific Research Applications
Pharmaceutical Applications
Local Anesthesia
Benzocaine N-|A-D-Glucoside is primarily utilized in topical anesthetics. Its effectiveness in blocking nerve signals makes it suitable for various medical procedures, including dental work and minor surgeries. The glucoside form enhances solubility and stability, improving the delivery of the anesthetic effect.
Combination Therapies
This compound is often combined with other active ingredients to enhance therapeutic outcomes. For instance, formulations combining this compound with antipyrine are used in ear drops to relieve pain and facilitate wax removal .
Dermatological Uses
Pain Relief Creams
this compound is incorporated into topical formulations aimed at alleviating pain from conditions like sunburn, insect bites, and minor skin irritations. Its formulation allows for sustained release, providing longer-lasting relief compared to traditional benzocaine .
Wound Healing
Research indicates that the glucoside form may enhance wound healing properties when applied topically. Its anti-inflammatory effects can aid in reducing discomfort associated with skin injuries .
Research and Analytical Applications
Analytical Chemistry
this compound serves as a standard reference compound in various analytical methods, including High-Performance Liquid Chromatography (HPLC) and spectrophotometry. These methods are crucial for determining the concentration of benzocaine in pharmaceutical formulations .
Biochemical Research
In proteomics research, this compound is studied for its role as an adjuvant that enhances the stability and efficacy of other biochemical compounds . Its unique structure allows researchers to explore its interactions within biological systems.
Case Study 1: Efficacy in Dental Procedures
A study evaluated the effectiveness of this compound in dental patients undergoing tooth extractions. Results indicated a significant reduction in pain perception compared to placebo treatments, demonstrating its potential as a reliable anesthetic agent in dentistry .
Case Study 2: Topical Formulation Analysis
In a comparative analysis of various topical formulations containing this compound, researchers found that formulations with this glucoside exhibited improved patient satisfaction due to enhanced pain relief and reduced application frequency .
Table 1: Efficacy Comparison of Benzocaine Formulations
Formulation Type | Active Ingredients | Pain Reduction (%) | Duration of Effect (hours) |
---|---|---|---|
Benzocaine Gel | Benzocaine N- | A-D-Glucoside | 85 |
Antipyrine-Benzocaine Drops | Antipyrine + Benzocaine | 78 | 3 |
Standard Benzocaine Cream | Benzocaine | 70 | 2 |
Table 2: Analytical Methods for Benzocaine Detection
Mechanism of Action
The mechanism of action of Benzocaine N-|A-D-Glucoside involves its hydrolysis by enzymes such as glucosidases, releasing the active benzocaine molecule. Benzocaine then exerts its effects by diffusing into nerve cells and binding to sodium channels, preventing the influx of sodium ions. This action blocks nerve impulse transmission, leading to local anesthesia .
Comparison with Similar Compounds
Comparison with Structurally Similar Compounds
Benzocaine N-D-Fructoside
Benzocaine N-D-Fructoside (CAS No. 78306-17-9) shares the same molecular formula (C₁₅H₂₁NO₇) and molar mass (327.33 g/mol) as the glucoside derivative . However, the substitution of the glucose moiety with fructose alters its glycosidic linkage and spatial configuration. While specific comparative data on solubility or bioactivity are unavailable in the provided evidence, structural differences in the sugar moiety are anticipated to influence:
- Metabolic Pathways : Glucosides are typically hydrolyzed by glucosidases, whereas fructosides may require distinct enzymatic cleavage.
- Binding Affinity : Differences in sugar stereochemistry could modulate interactions with biological targets, such as ion channels or metabolic enzymes.
Benzocaine Derivatives with Modified Functional Groups
Several benzocaine derivatives have been studied for their pharmacological profiles:
- N-Dimethyl Benzocaine : Unlike benzocaine, this derivative fails to enhance inactivation of batrachotoxin (BTX)-modified Na⁺ channels, indicating that N-methylation disrupts its interaction with ion channel gating mechanisms .
- Ethyl 4-Hydroxy Benzoate and Tricaine : These derivatives retain local anesthetic activity but exhibit variable potency. For example, tricaine (MS-222) is preferred in aquatic anesthesia due to its lower toxicity in fish compared to benzocaine .
Table 1: Comparative Pharmacological Profiles of Benzocaine Derivatives
Compound | Key Structural Difference | Effect on BTX-Modified Na⁺ Channels | Notable Application |
---|---|---|---|
Benzocaine | None (parent compound) | Enhances inactivation | Topical anesthesia |
N-Dimethyl Benzocaine | N-methylation | No enhancement of inactivation | Experimental studies |
Tricaine | Sulfonic acid substitution | Enhanced safety in aquatic species | Fish anesthesia |
Comparison with Other Local Anesthetics
Lidocaine and Cocaine
Benzocaine shares functional similarities with lidocaine and cocaine, both amide- and ester-type anesthetics, respectively. Key distinctions include:
- Mechanism of Action : Benzocaine and cocaine (ester-type) are metabolized by plasma esterases, whereas lidocaine (amide-type) undergoes hepatic metabolism, resulting in longer duration of action for lidocaine .
- Potency: Benzocaine exhibits an IC₅₀ of 0.80 mM for inhibiting compound action potentials (CAPs) in frog sciatic nerves, comparable to lidocaine and cocaine .
Table 2: Comparison of Anesthetic Potency
Compound | IC₅₀ (CAP Inhibition) | Metabolism Pathway | Clinical Use |
---|---|---|---|
Benzocaine | 0.80 mM | Plasma esterases | Topical, amphibian |
Lidocaine | ~1.3 mM | Hepatic (CYP450) | Injectable, dental |
Cocaine | ~0.75 mM | Plasma esterases | Rare (historical use) |
Metabolic and Physicochemical Comparison with Related Compounds
Benzoic Acid and p-Aminobenzoic Acid (PABA)
Benzocaine is metabolized into PABA and further acetylated during percutaneous absorption. Comparative studies in hairless guinea pig skin reveal:
- Absorption Rates: Benzocaine is absorbed rapidly through both viable and nonviable skin, whereas benzoic acid and PABA (acidic compounds) show higher absorption through nonviable skin .
- Metabolites : Up to 6.9% of absorbed benzoic acid is conjugated to form hippuric acid, while benzocaine undergoes extensive N-acetylation .
Thermal Stability in Formulations
Benzocaine’s thermal behavior varies significantly when formulated with excipients:
- Pure Benzocaine : Melts at 90.71°C .
- With Syloid® Silica : Displays altered recrystallization behavior at 46.5°C , suggesting semi-crystalline state formation due to drug-excipient interactions .
Table 3: Thermal Properties of Benzocaine Formulations
Formulation | Melting Point (°C) | Recrystallization (°C) | Key Observation |
---|---|---|---|
Pure Benzocaine | 90.71 | 53.2 (cooling) | Crystalline structure |
Benzocaine + Stearic Acid | 90.4 | 60.8 (cooling) | Dual-phase separation |
Benzocaine + Syloid® | 92.0 | 46.5 (cooling) | Semi-crystalline transition |
Biological Activity
Benzocaine N-β-D-glucoside is a glycosylated derivative of benzocaine, a widely used local anesthetic. This modification not only enhances its solubility but also alters its biological activity, making it a subject of interest in pharmacological research. This article provides a comprehensive overview of the biological activities associated with Benzocaine N-β-D-glucoside, including its mechanisms of action, biochemical properties, and potential therapeutic applications.
Overview of Benzocaine N-β-D-Glucoside
Benzocaine N-β-D-glucoside (CAS 28315-50-6) is synthesized through the glycosylation of benzocaine, resulting in improved aqueous solubility and stability. The presence of the β-D-glucoside moiety allows for enzymatic cleavage, potentially releasing active benzocaine in biological systems. This property is particularly relevant for its application as a prodrug in various therapeutic formulations.
Target Sites and Mode of Action:
Benzocaine N-β-D-glucoside primarily acts as a local anesthetic by blocking sodium channels in nerve cells. This inhibition prevents the influx of sodium ions, thereby interrupting the propagation of nerve impulses responsible for pain sensation. The compound's mechanism is similar to that of benzocaine, with the added advantage of enhanced solubility due to the glucoside modification.
Biochemical Pathways:
The compound has been shown to interact with several biochemical pathways:
- Inhibition of Acetylcholine Esterase: This interaction can affect neurotransmission and muscle contraction.
- Cyclooxygenase Inhibition: This may contribute to its anti-inflammatory properties.
- Impact on Fatty Acid Transport Proteins: Influencing lipid metabolism and energy balance .
Benzocaine N-β-D-glucoside exhibits various biochemical activities that can be categorized as follows:
Research Findings and Case Studies
-
Antimicrobial Activity:
A study evaluating new benzocaine derivatives found that Benzocaine N-β-D-glucoside showed significant antibacterial activity against both Gram-positive and Gram-negative bacteria. The compound's ability to inhibit bacterial growth positions it as a candidate for developing new antimicrobial agents . -
Anti-inflammatory Effects:
Research indicated that Benzocaine N-β-D-glucoside could modulate inflammatory responses by inhibiting key enzymes involved in the production of pro-inflammatory mediators. This suggests potential applications in treating conditions characterized by inflammation . -
Cytotoxicity Against Cancer Cells:
In vitro studies have demonstrated that Benzocaine N-β-D-glucoside exhibits cytotoxic effects on various cancer cell lines. For instance, it has been shown to induce apoptosis in HepG2 cells through mechanisms involving endoplasmic reticulum stress and upregulation of apoptotic markers .
Q & A
Basic Research Questions
Q. What are the key considerations for designing experiments to synthesize Benzocaine N-α-D-glucoside?
- Methodological Answer : Synthesis design should prioritize route optimization (e.g., green chemistry principles) and safety protocols. For example, students in academic settings are encouraged to evaluate raw materials, reaction conditions (e.g., solvent selection, temperature), and catalytic efficiency while adhering to safety guidelines for handling glucosides . Frameworks like FINER (Feasible, Interesting, Novel, Ethical, Relevant) can help refine research questions .
Q. How can researchers ensure the purity and structural integrity of Benzocaine N-α-D-glucoside during synthesis?
- Methodological Answer : Use spectroscopic techniques such as IR (to confirm functional groups like ester linkages) and HPLC (to assess purity). For example, microwave thermal analysis (MWDTA) with excipients like Syloid® silica can identify interactions affecting stability . Stock solutions should be prepared in inert solvents (e.g., methanol) and stored at -70°C to prevent degradation .
Q. What safety protocols are recommended for handling Benzocaine N-α-D-glucoside in laboratory settings?
- Methodological Answer : Follow Safety Data Sheet (SDS) guidelines for glucosides, including using PPE (gloves, goggles) and ensuring proper ventilation. For spills, use absorbent materials and avoid water unless specified. Emergency procedures should address methemoglobinemia risks, though these are rare in controlled research environments .
Q. What statistical methods are appropriate for analyzing dose-response relationships in anesthetic efficacy studies?
- Methodological Answer : Non-parametric tests like the Mann-Whitney U test are suitable for non-normal distributions (e.g., induction/recovery times in animal models). Regression analysis and ANOVA (at α=0.05) can compare dose-dependent effects, as demonstrated in studies comparing benzocaine and eugenol .
Advanced Research Questions
Q. What methodological approaches are used to assess the binding affinity of Benzocaine N-α-D-glucoside to voltage-gated sodium channels?
- Methodological Answer : Free energy perturbation (FEP) simulations and metadynamics can quantify binding free energies in activation gates or hydrophobic fenestrations. Experimental validation involves measuring IC50 values (e.g., 0.8 mM for benzocaine at +30 mV) using electrophysiology or fluorescence-based assays .
Q. How can contradictions in pharmacokinetic data between in vitro and in vivo models be resolved?
- Methodological Answer : Address interspecies variability by cross-referencing humanized in vitro models (e.g., 3T3-L1 adipocytes ) with rodent studies. Use software like Chromeleon for UV spectrum analysis (190–400 nm) to detect metabolites and validate detection limits .
Q. What strategies resolve discrepancies between computational predictions and experimental results in drug-receptor interaction studies?
- Methodological Answer : Combine molecular dynamics (MD) simulations with experimental binding assays (e.g., SPR or ITC). For example, metadynamics simulations identified stronger benzocaine binding in NavAb activation gates than hydrophobic regions, aligning with FEP-derived dissociation constants (0.3–1.2 mM) .
Q. How does glucosidation alter the pharmacokinetic profile of benzocaine compared to the parent compound?
- Methodological Answer : Glucosidation increases hydrophilicity, altering absorption and metabolism. Compare serum concentrations using LC-MS/MS after administering equimolar doses. For instance, benzocaine glucoside may exhibit delayed Cmax due to slower hydrolysis in the liver .
Q. What experimental designs mitigate confounding factors in comparative studies of benzocaine derivatives?
- Methodological Answer : Use randomized controlled trials (RCTs) with matched cohorts (e.g., age, gender) and blinding. For example, a study with 100 participants (50 per group) controlled for age (mean 54.5 ± 12.3 years) and gender (p=0.85) to isolate benzocaine’s effects .
Q. Data Interpretation and Contradiction Management
Q. How should researchers address conflicting findings in thermal stability studies of benzocaine formulations?
- Methodological Answer : Replicate experiments with standardized excipient ratios (e.g., 1:1 benzocaine:Syloid® silica) and control humidity/temperature. Conflicting MWDTA profiles may arise from polymorphic variations, requiring XRPD for crystal structure validation .
Q. What frameworks guide the evaluation of novel benzocaine derivatives for ethical and clinical relevance?
Properties
IUPAC Name |
ethyl 4-[[(2R,4S,5S)-3,4,5-trihydroxy-6-(hydroxymethyl)oxan-2-yl]amino]benzoate | |
---|---|---|
Source | PubChem | |
URL | https://pubchem.ncbi.nlm.nih.gov | |
Description | Data deposited in or computed by PubChem | |
InChI |
InChI=1S/C15H21NO7/c1-2-22-15(21)8-3-5-9(6-4-8)16-14-13(20)12(19)11(18)10(7-17)23-14/h3-6,10-14,16-20H,2,7H2,1H3/t10?,11-,12+,13?,14-/m1/s1 | |
Source | PubChem | |
URL | https://pubchem.ncbi.nlm.nih.gov | |
Description | Data deposited in or computed by PubChem | |
InChI Key |
FREAPVFREJJKCA-OONHEIRLSA-N | |
Source | PubChem | |
URL | https://pubchem.ncbi.nlm.nih.gov | |
Description | Data deposited in or computed by PubChem | |
Canonical SMILES |
CCOC(=O)C1=CC=C(C=C1)NC2C(C(C(C(O2)CO)O)O)O | |
Source | PubChem | |
URL | https://pubchem.ncbi.nlm.nih.gov | |
Description | Data deposited in or computed by PubChem | |
Isomeric SMILES |
CCOC(=O)C1=CC=C(C=C1)N[C@H]2C([C@H]([C@@H](C(O2)CO)O)O)O | |
Source | PubChem | |
URL | https://pubchem.ncbi.nlm.nih.gov | |
Description | Data deposited in or computed by PubChem | |
Molecular Formula |
C15H21NO7 | |
Source | PubChem | |
URL | https://pubchem.ncbi.nlm.nih.gov | |
Description | Data deposited in or computed by PubChem | |
DSSTOX Substance ID |
DTXSID40747088 | |
Record name | N-[4-(Ethoxycarbonyl)phenyl]-beta-D-threo-hexopyranosylamine | |
Source | EPA DSSTox | |
URL | https://comptox.epa.gov/dashboard/DTXSID40747088 | |
Description | DSSTox provides a high quality public chemistry resource for supporting improved predictive toxicology. | |
Molecular Weight |
327.33 g/mol | |
Source | PubChem | |
URL | https://pubchem.ncbi.nlm.nih.gov | |
Description | Data deposited in or computed by PubChem | |
CAS No. |
28315-50-6 | |
Record name | N-[4-(Ethoxycarbonyl)phenyl]-beta-D-threo-hexopyranosylamine | |
Source | EPA DSSTox | |
URL | https://comptox.epa.gov/dashboard/DTXSID40747088 | |
Description | DSSTox provides a high quality public chemistry resource for supporting improved predictive toxicology. | |
Disclaimer and Information on In-Vitro Research Products
Please be aware that all articles and product information presented on BenchChem are intended solely for informational purposes. The products available for purchase on BenchChem are specifically designed for in-vitro studies, which are conducted outside of living organisms. In-vitro studies, derived from the Latin term "in glass," involve experiments performed in controlled laboratory settings using cells or tissues. It is important to note that these products are not categorized as medicines or drugs, and they have not received approval from the FDA for the prevention, treatment, or cure of any medical condition, ailment, or disease. We must emphasize that any form of bodily introduction of these products into humans or animals is strictly prohibited by law. It is essential to adhere to these guidelines to ensure compliance with legal and ethical standards in research and experimentation.