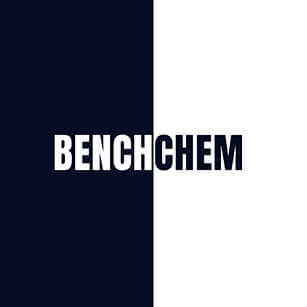
Irbesartan dimer impurity
- Click on QUICK INQUIRY to receive a quote from our team of experts.
- With the quality product at a COMPETITIVE price, you can focus more on your research.
Overview
Description
Irbesartan dimer impurity is a process-related degradation product formed during the synthesis or storage of the angiotensin II receptor blocker (ARB) drug irbesartan. Structurally, it arises from the covalent linkage of two irbesartan molecules, typically via reactions involving reactive intermediates such as brominated precursors or oxidative coupling . Analytical methods like reverse-phase high-performance liquid chromatography (RP-HPLC) with photodiode array (PDA) detection have been validated to identify and quantify this impurity, with limits of detection (LOD) and quantification (LOQ) established at 16–18 µg/mL and 48–56 µg/mL, respectively .
Preparation Methods
Structural and Mechanistic Basis of Irbesartan Impurities
Chemical Identity of Impurity A
Irbesartan impurity A is a hydrolytic degradation product formed under alkaline conditions. Its structure arises from the cleavage of the tetrazole-protecting trityl group and subsequent side reactions during synthesis (Fig. 1). The compound is characterized by a cyclopentane core substituted with valeramide and biphenyl-tetrazole moieties .
Synthetic Methodologies for Irbesartan Impurity A
Stepwise Synthesis from N-(Triphenylmethyl)-5-(4'-Bromomethylbiphenyl-2-yl) Tetrazole
The patented method involves two stages (Table 1):
Table 1: Synthesis of Irbesartan Impurity A
Step | Reagents/Conditions | Purpose | Yield |
---|---|---|---|
1 | N-(Trityl)-5-(4'-bromomethylbiphenyl-2-yl) tetrazole, NaOH (32%), TBAB, CH₂Cl₂, 35°C, 10h | Alkaline hydrolysis and coupling with 1-(valeramido)cyclopentane carboxamide | 85–90% |
2 | Tetrahydrofuran, C1–C5 alcohol, acetyl chloride, acetone/hexane recrystallization | Trityl deprotection and purification | 70–75% |
Alkaline Hydrolysis and Coupling
The first step employs tetrabutylammonium bromide (TBAB) as a phase-transfer catalyst to facilitate the reaction between the bromomethyl intermediate and 1-(valeramido)cyclopentane carboxamide in dichloromethane. Sodium hydroxide (32%) drives the nucleophilic substitution, forming the trityl-protected precursor .
Trityl Deprotection and Purification
Deprotection using acetyl chloride in low-water alcohols (e.g., methanol) removes the trityl group, yielding impurity A. Recrystallization with acetone/hexane (1:1.5–1:3 ratio) achieves >99% purity, as confirmed by HPLC (Fig. 2) .
Critical Process Parameters
-
Temperature control : Reactions performed at 10–35°C prevent undesired side reactions.
-
Solvent selection : Dichloromethane and tetrahydrofuran optimize solubility, while acetone/hexane mixtures enhance crystal lattice formation.
-
Moisture content : <3% water in deprotection steps minimizes hydrolysis of the tetrazole ring .
Analytical Characterization and Validation
Chromatographic Purity Assessment
The patents validate impurity A using a reversed-phase HPLC method:
-
Column : C18 (250 × 4.6 mm, 5 µm)
-
Mobile phase : Phosphoric acid (pH 3.2)/acetonitrile (62:38)
-
Detection : UV at 220 nm
-
Retention time : 8.2 min, matching the USP reference standard (Fig. 1–2) .
Table 2: Purity Analysis of Impurity A
Batch | Purity (%) | Related Substances (%) |
---|---|---|
1 | 99.3 | 0.7 |
2 | 99.1 | 0.9 |
3 | 99.5 | 0.5 |
Spectroscopic Confirmation
-
UV-Vis : λₘₐₓ at 220 nm, consistent with the tetrazole chromophore.
-
Mass spectrometry : m/z 577.2 [M+H]⁺, confirming the molecular formula C₃₀H₃₂N₆O₂ .
Challenges and Best Practices in Impurity Preparation
Scaling and Reproducibility
-
Microfluidic reactors : Patent data suggest batch processes, but continuous-flow systems could improve yield consistency for gram-scale synthesis.
-
In-process controls : Real-time HPLC monitoring ensures reaction completeness during deprotection and crystallization .
Regulatory Considerations
Chemical Reactions Analysis
Irbesartan dimer impurity can undergo various chemical reactions, including:
Oxidation: The dimer impurity can be oxidized under specific conditions, leading to the formation of different oxidation products.
Reduction: Reduction reactions can break down the dimer impurity into smaller fragments.
Substitution: The dimer impurity can undergo substitution reactions, where functional groups are replaced by other groups under suitable conditions.
Common reagents used in these reactions include oxidizing agents like hydrogen peroxide, reducing agents like sodium borohydride, and various catalysts to facilitate substitution reactions. The major products formed depend on the specific reaction conditions and reagents used .
Scientific Research Applications
Synthesis and Characterization
The synthesis of irbesartan dimer impurity typically involves various chemical reactions that can lead to its formation as a secondary product during the manufacturing process of irbesartan. The methods for synthesizing this impurity have been documented in several patents, detailing processes such as:
- Alkaline Hydrolysis : Involves treating irbesartan under alkaline conditions to facilitate the formation of the dimer impurity .
- Liquid Chromatography : High-performance liquid chromatography (HPLC) is often employed to analyze and purify the dimer impurity, ensuring that it meets specified purity standards .
The characterization of this compound is crucial for understanding its chemical properties and behavior in biological systems. Techniques such as mass spectrometry and nuclear magnetic resonance (NMR) spectroscopy are commonly used to elucidate its structure and confirm its identity.
Research on the biological activity of this compound is essential for assessing its potential impact on human health. Studies have focused on:
- Toxicological Assessments : Evaluating the toxicity profile of the dimer impurity to determine its safety when present in pharmaceutical formulations. This includes investigating cytotoxic effects on various cell lines and potential interactions with biological targets.
- Pharmacological Effects : Understanding whether the dimer exhibits any pharmacological activity that could influence the therapeutic outcomes of irbesartan treatment. This involves studying its effects on blood pressure regulation and other cardiovascular parameters .
Regulatory Significance
The presence of impurities like irbesartan dimer is a critical concern in pharmaceutical manufacturing due to regulatory requirements set by agencies such as the FDA and EMA. The following aspects are particularly noteworthy:
- Quality by Design (QbD) : Implementing QbD principles allows manufacturers to identify critical quality attributes (CQA) related to dimer impurities during production. This approach helps in minimizing variability and ensuring consistent product quality .
- Compliance with Standards : Regulatory bodies require comprehensive impurity profiling to ensure that drug products meet safety standards. The acceptable limits for impurities are established based on their potential impact on patient health .
Industrial Applications
In the pharmaceutical industry, understanding and controlling this compound is vital for:
- Quality Control : Regular monitoring of impurity levels during production processes helps maintain product integrity and compliance with pharmacopoeial standards.
- Formulation Development : Knowledge about the stability and degradation pathways of the dimer impurity informs formulation strategies to enhance the shelf-life and efficacy of irbesartan-containing products .
Case Studies
Several case studies illustrate the significance of managing this compound:
- Manufacturing Variability : A study highlighted that variations in reaction time during synthesis could lead to significant fluctuations in dimer content, impacting yield and quality control measures .
- Toxicology Evaluation : Research demonstrated that certain concentrations of this compound exhibited cytotoxic effects on specific cell lines, prompting further investigation into acceptable exposure levels in pharmaceutical formulations.
Mechanism of Action
The mechanism of action of Irbesartan dimer impurity is not well-defined, as it is primarily a byproduct rather than an active pharmaceutical ingredient. understanding its formation and degradation pathways is essential for ensuring the purity and safety of Irbesartan. The impurity may interact with molecular targets and pathways involved in the synthesis and stability of the drug .
Comparison with Similar Compounds
Structural and Functional Similarities
Irbesartan dimer impurity shares structural homology with other sartan-class impurities, including valsartan acid , losartan oxidative byproducts , and candesartan derivatives (Table 1). These compounds retain the tetrazole biphenylmethyl backbone but differ in substituents and degradation pathways:
- Valsartan acid : A primary metabolite of valsartan, formed via hydroxyl radical-mediated oxidation during ozonation (kO3 ≈ 34 M⁻¹s⁻¹) .
- Losartan oxidative impurities: Exhibit reduced sensor sensitivity (5× lower than irbesartan) due to minor structural variations in the imidazole ring .
- Candesartan : Demonstrates environmental persistence despite structural similarity, contrasting with irbesartan and valsartan, which degrade readily in riverbank filtration systems .
Table 1: Key Characteristics of Sartan-Class Impurities
*Calculated based on and synthesis pathways .
Stability and Degradation Behavior
- Irbesartan dimer : Exhibits stability under acidic and basic stress conditions, with homogeneous degradation peaks confirmed by PDA .
- Valsartan acid : Degrades faster than irbesartan during ozonation (kO3 = 23–34 M⁻¹s⁻¹) due to electron-deficient tetrazole groups .
- N-Nitrosoirbesartan: A genotoxic impurity formed under nitrosating conditions, structurally distinct from the dimer but critical for regulatory control .
Analytical Challenges
- Cross-reactivity: Molecularly imprinted polymer (MIP) sensors show non-specific responses to sartans, complicating selective detection .
- Chromatographic separation: RP-HPLC methods resolve irbesartan dimer from other impurities (e.g., 2-cyano-4'-bromomethyl biphenyl) using isocratic mobile phases .
Biological Activity
Irbesartan dimer impurity, identified by the CAS number 1346598-52-4, is a byproduct of the synthesis of Irbesartan, an angiotensin II receptor antagonist used primarily for treating hypertension and diabetic nephropathy. Understanding the biological activity of this impurity is crucial for ensuring the safety and efficacy of Irbesartan formulations. This article delves into the biological activity, synthesis, and implications of this compound based on diverse research findings.
Chemical Structure and Synthesis
This compound can be chemically described as follows:
Property | Details |
---|---|
Molecular Formula | C39H38N10O |
Molecular Weight | 662.8 g/mol |
Synonyms | 4’-[2-[1-[(2’-tetrazoylbiphen-4-yl)methyl]-4-oxo-1,3-diazaspiro[4,4]non-2-en-2-yl]pentyl]biphenyl-2-tetrazole |
The synthesis of Irbesartan involves several steps, during which various impurities can form, including the dimer impurity. The formation typically occurs during condensation reactions and tetrazole formation steps. The presence of this impurity is critical for pharmaceutical quality control, as it can affect drug stability and safety .
While Irbesartan functions by blocking the action of angiotensin II to lower blood pressure, the specific biological activity of the dimer impurity is less well-defined. It is primarily considered a byproduct rather than an active pharmaceutical ingredient. However, understanding its formation and degradation pathways is essential for ensuring the purity and safety of Irbesartan .
Biological Activity and Toxicity Studies
Research into the biological activity of this compound has focused on its potential toxicity and interaction with biological systems. Some key findings include:
- Toxicological Assessments : Studies have indicated that impurities in pharmaceuticals can lead to adverse effects, including genotoxicity. The dimer impurity's potential toxic effects are evaluated through in vitro assays that assess cell viability and mutagenicity .
- Pharmacokinetics : The pharmacokinetic profile of the impurity may differ from that of Irbesartan itself. Research aims to understand how this impurity behaves in biological systems, including absorption, distribution, metabolism, and excretion (ADME) characteristics .
Case Studies
- Analytical Characterization : A study employed advanced analytical techniques such as HPLC and LC-MS to characterize this compound in pharmaceutical formulations. This research highlighted the importance of monitoring impurity levels to comply with regulatory standards set by organizations like the FDA and ICH .
- Environmental Impact : Another investigation explored the environmental fate of Irbesartan and its impurities when subjected to various treatment methods. The study found that the dimer impurity could persist in certain conditions, raising concerns about its long-term effects on ecosystems .
Q & A
Basic Questions
Q. What analytical methods are recommended for detecting and quantifying Irbesartan dimer impurities?
A reversed-phase HPLC method using a Kromasil C18 column (250 × 4.6 mm, 5 µm) with a mobile phase of pH 3.2 buffer and acetonitrile (60:40 v/v) at 1.5 mL/min flow rate is widely validated for detecting dimer impurities like 2-cyano-4’-bromomethyl biphenyl. Detection is performed at 258 nm with a column temperature of 40°C. This method achieves a limit of quantification (LOQ) of 0.506 µg/g and demonstrates high precision (%RSD <2%) .
Q. How are genotoxic impurities in Irbesartan, such as 2-cyano-4’-bromomethyl biphenyl, identified and regulated?
Genotoxic impurities are identified using chromatographic methods (e.g., HPLC, LC-MS/MS) coupled with structural validation via NMR or MS. Regulatory limits are based on the Threshold of Toxicological Concern (TTC), with a maximum allowable limit of 5.00 µg/g for 2-cyano-4’-bromomethyl biphenyl, as per ICH M7 and EMA guidelines .
Q. What distinguishes dimer impurities from other process-related impurities in Irbesartan synthesis?
Dimer impurities arise from condensation or nucleophilic reactions during synthesis, such as acid-catalyzed dimerization of intermediates. They are structurally distinct from monomers and require separation via specificity testing (e.g., resolution >2.0 between impurity and API peaks in HPLC). For example, 2-cyano-4’-bromomethyl biphenyl is a synthesis intermediate dimer, whereas azide impurities originate from reagent residues .
Q. Which regulatory bodies define acceptance criteria for Irbesartan impurities, and what are the key thresholds?
The ICH Q3A/B, EMA, and USP guidelines set impurity thresholds. For example, the total impurities in Irbesartan must not exceed 0.6%, with individual impurities (e.g., dimer-related compounds) capped at 0.2%. Genotoxic impurities like 2-cyano-4’-bromomethyl biphenyl are restricted to 5.00 µg/g .
Q. How is the specificity of an analytical method confirmed for Irbesartan dimer impurity analysis?
Specificity is validated by spiking the API with impurities and demonstrating baseline separation in chromatograms. Forced degradation studies (acid/base hydrolysis, oxidation) ensure the method can resolve degradation products from the dimer impurity. Overlay chromatograms of stressed samples and spiked batches are compared to confirm no co-elution .
Advanced Research Questions
Q. What parameters are critical for validating an HPLC method to quantify trace-level dimer impurities?
Key parameters include:
- Linearity : Correlation coefficient (R²) ≥0.999 over 50–150% of the evaluation limit .
- Precision : Intra-day and inter-day %RSD <2% for replicate injections .
- Accuracy : Recovery of 98–103% via spiking at LOQ, 50%, 100%, and 150% levels .
- Robustness : Method stability under altered flow rates (±0.1 mL/min) and column temperatures (±5°C) .
Q. How can researchers resolve discrepancies in stability data for Irbesartan dimer impurities?
Discrepancies often arise from matrix effects or degradation pathways. Use forced degradation studies to identify instability triggers (e.g., light, pH). For example, solution stability tests show 2-cyano-4’-bromomethyl biphenyl remains stable in acetonitrile diluent for 24 hours at room temperature. Conflicting data should be cross-validated with LC-MS/MS to rule out degradation artifacts .
Q. What process modifications reduce dimer impurity formation during Irbesartan synthesis?
Optimizing reaction stoichiometry, temperature, and catalyst use minimizes dimerization. For example, controlling bromomethylation step pH and avoiding excess reagents reduces 2-cyano-4’-bromomethyl biphenyl formation. Post-synthesis purification via crystallization or chromatography further lowers impurity levels .
Q. How does LC-MS/MS compare to HPLC for quantifying trace azide impurities in Irbesartan?
LC-MS/MS offers higher sensitivity (LOQ <0.1 µg/g) and selectivity for azides like AZBT, which are carcinogenic. In contrast, HPLC-UV is cost-effective but less sensitive (LOQ ~0.3 µg/g). For regulatory compliance, LC-MS/MS is preferred for azides, while HPLC suffices for non-volatile dimers .
Q. What strategies address nitrosamine impurity risks in Irbesartan, and how are they justified to regulators?
Risk assessment includes evaluating amine precursors and nitrosating agents. If synthesis of a nitrosamine is theoretically impossible (e.g., lacking amine groups), confirmatory testing may be waived. Justification requires structural analysis, reactivity studies, and literature evidence, as per EMA Article 5(3) guidelines .
Properties
CAS No. |
1346598-52-4 |
---|---|
Molecular Formula |
C39H38N10O |
Molecular Weight |
662.8 g/mol |
IUPAC Name |
3-[[4-[2-(2H-tetrazol-5-yl)phenyl]phenyl]methyl]-2-[1-[4-[2-(2H-tetrazol-5-yl)phenyl]phenyl]pentan-2-yl]-1,3-diazaspiro[4.4]non-1-en-4-one |
InChI |
InChI=1S/C39H38N10O/c1-2-9-30(24-26-14-18-28(19-15-26)31-10-3-5-12-33(31)35-41-45-46-42-35)37-40-39(22-7-8-23-39)38(50)49(37)25-27-16-20-29(21-17-27)32-11-4-6-13-34(32)36-43-47-48-44-36/h3-6,10-21,30H,2,7-9,22-25H2,1H3,(H,41,42,45,46)(H,43,44,47,48) |
InChI Key |
WRDCNWPENKTBFX-UHFFFAOYSA-N |
Canonical SMILES |
CCCC(CC1=CC=C(C=C1)C2=CC=CC=C2C3=NNN=N3)C4=NC5(CCCC5)C(=O)N4CC6=CC=C(C=C6)C7=CC=CC=C7C8=NNN=N8 |
Purity |
> 95% |
quantity |
Milligrams-Grams |
Synonyms |
4’-[2-[1-[(2’-tetrazoylbiphen-4-yl)methyl]-4-oxo-1,3-diazaspiro[4,4]non-2-en-2-yl]pentyl]biphenyl-2-tetrazole; |
Origin of Product |
United States |
Disclaimer and Information on In-Vitro Research Products
Please be aware that all articles and product information presented on BenchChem are intended solely for informational purposes. The products available for purchase on BenchChem are specifically designed for in-vitro studies, which are conducted outside of living organisms. In-vitro studies, derived from the Latin term "in glass," involve experiments performed in controlled laboratory settings using cells or tissues. It is important to note that these products are not categorized as medicines or drugs, and they have not received approval from the FDA for the prevention, treatment, or cure of any medical condition, ailment, or disease. We must emphasize that any form of bodily introduction of these products into humans or animals is strictly prohibited by law. It is essential to adhere to these guidelines to ensure compliance with legal and ethical standards in research and experimentation.