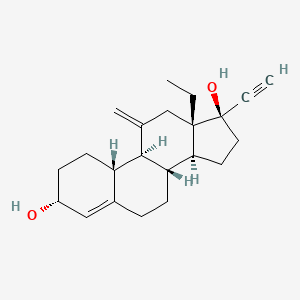
3alpha-Hydroxydesogestrel
Overview
Description
3alpha-Hydroxydesogestrel is a metabolite of desogestrel, a synthetic progestin used in hormonal contraceptives. It is a steroid with the chemical formula C22H30O2 and a molecular weight of 326.47 g/mol . This compound is known for its role in the metabolism of desogestrel and its involvement in various biochemical pathways.
Mechanism of Action
Target of Action
3alpha-Hydroxydesogestrel, a synthetic progestin, primarily targets the progesterone receptor . The progesterone receptor plays a crucial role in the regulation of the menstrual cycle and maintenance of pregnancy .
Mode of Action
This compound enters the cell passively and binds selectively to the progesterone receptor, generating low androgenic activity . This binding produces an effect similar to a transcription factor, leading to modifications in the mRNA synthesis .
Biochemical Pathways
The primary biochemical pathway affected by this compound involves the conversion of desogestrel to its active metabolite, etonogestrel . This conversion is facilitated by the hydroxylation at the C3 position of the desogestrel molecule .
Pharmacokinetics
This compound is rapidly metabolized in the intestinal mucosa and liver to form etonogestrel, its biologically active metabolite . The pharmacokinetic parameters of etonogestrel are generally comparable with those of levonorgestrel and norethindrone . Any differences in pharmacologic activities must be attributed to differences in intrinsic activities .
Result of Action
The main therapeutic effect of this compound is related to the inhibition of ovulation in 97% of the cycles . This effect was proven in clinical trials in non-breastfeeding women, from which the Pearl failure rate was reported to be 0.17 per 100 women-years .
Biochemical Analysis
Biochemical Properties
3alpha-Hydroxydesogestrel plays a role in the metabolism of steroids and non-steroidal compounds in humans and other species . This enzyme catalyzes the chemical reaction of conversion of 3-ketosteroids into 3alpha-hydroxysteroids .
Cellular Effects
Desogestrel, from which this compound is derived, has been shown to have potent functional antiestrogenic effects in certain tissues . It dose-dependently antagonizes the effects of ethinylestradiol on the vaginal epithelium, cervical mucus, and endometrium .
Molecular Mechanism
Desogestrel enters the cell passively and acts by binding selectively to the progesterone receptor and generating low androgenic activity . Its binding produces an effect like a transcription factor and thus, it produces modifications in the mRNA synthesis .
Dosage Effects in Animal Models
Animal models play a crucial role in advancing biomedical research, especially in the field of gene therapy .
Metabolic Pathways
Desogestrel is rapidly metabolized in the intestinal mucosa and by first-pass hepatic metabolism to form the major metabolite of desogestrel, which is etonogestrel . This modification is described by the hydroxylation in C3 of the desogestrel molecule .
Subcellular Localization
Prediction of subcellular localization of proteins from their amino acid sequences has a long history in bioinformatics and is still actively developing .
Preparation Methods
3alpha-Hydroxydesogestrel is typically synthesized from desogestrel through enzymatic reactions. The primary method involves the use of 3alpha-hydroxysteroid dehydrogenase, which catalyzes the conversion of desogestrel to this compound . The reaction conditions usually require the presence of NADPH as a cofactor
Chemical Reactions Analysis
3alpha-Hydroxydesogestrel undergoes several types of chemical reactions, including:
Oxidation: This reaction involves the conversion of the hydroxyl group to a ketone group, often using oxidizing agents such as potassium permanganate or chromium trioxide.
Reduction: The compound can be reduced back to desogestrel using reducing agents like sodium borohydride or lithium aluminum hydride.
Substitution: Various substitution reactions can occur at the hydroxyl group, leading to the formation of different derivatives.
Common reagents and conditions used in these reactions include organic solvents like methanol or ethanol, and reaction temperatures typically range from room temperature to 100°C. Major products formed from these reactions include oxidized or reduced forms of the compound, as well as various substituted derivatives.
Scientific Research Applications
3alpha-Hydroxydesogestrel has several scientific research applications, including:
Comparison with Similar Compounds
3alpha-Hydroxydesogestrel is similar to other hydroxylated metabolites of progestins, such as 3beta-Hydroxydesogestrel and etonogestrel . it is unique in its specific hydroxylation pattern and its distinct metabolic profile. Compared to desogestrel, this compound has different binding affinities and biological activities, making it a valuable compound for studying the metabolism and function of progestins .
Properties
IUPAC Name |
(3R,8S,9S,10R,13S,14S,17R)-13-ethyl-17-ethynyl-11-methylidene-1,2,3,6,7,8,9,10,12,14,15,16-dodecahydrocyclopenta[a]phenanthrene-3,17-diol | |
---|---|---|
Source | PubChem | |
URL | https://pubchem.ncbi.nlm.nih.gov | |
Description | Data deposited in or computed by PubChem | |
InChI |
InChI=1S/C22H30O2/c1-4-21-13-14(3)20-17-9-7-16(23)12-15(17)6-8-18(20)19(21)10-11-22(21,24)5-2/h2,12,16-20,23-24H,3-4,6-11,13H2,1H3/t16-,17+,18+,19+,20-,21+,22+/m1/s1 | |
Source | PubChem | |
URL | https://pubchem.ncbi.nlm.nih.gov | |
Description | Data deposited in or computed by PubChem | |
InChI Key |
ZMLDTNLDYRJTAZ-JASYKLOUSA-N | |
Source | PubChem | |
URL | https://pubchem.ncbi.nlm.nih.gov | |
Description | Data deposited in or computed by PubChem | |
Canonical SMILES |
CCC12CC(=C)C3C(C1CCC2(C#C)O)CCC4=CC(CCC34)O | |
Source | PubChem | |
URL | https://pubchem.ncbi.nlm.nih.gov | |
Description | Data deposited in or computed by PubChem | |
Isomeric SMILES |
CC[C@]12CC(=C)[C@H]3[C@H]([C@@H]1CC[C@]2(C#C)O)CCC4=C[C@@H](CC[C@H]34)O | |
Source | PubChem | |
URL | https://pubchem.ncbi.nlm.nih.gov | |
Description | Data deposited in or computed by PubChem | |
Molecular Formula |
C22H30O2 | |
Source | PubChem | |
URL | https://pubchem.ncbi.nlm.nih.gov | |
Description | Data deposited in or computed by PubChem | |
Molecular Weight |
326.5 g/mol | |
Source | PubChem | |
URL | https://pubchem.ncbi.nlm.nih.gov | |
Description | Data deposited in or computed by PubChem | |
CAS No. |
70805-84-4 | |
Record name | 3alpha-Hydroxydesogestrel | |
Source | ChemIDplus | |
URL | https://pubchem.ncbi.nlm.nih.gov/substance/?source=chemidplus&sourceid=0070805844 | |
Description | ChemIDplus is a free, web search system that provides access to the structure and nomenclature authority files used for the identification of chemical substances cited in National Library of Medicine (NLM) databases, including the TOXNET system. | |
Record name | 3.ALPHA.-HYDROXYDESOGESTREL | |
Source | FDA Global Substance Registration System (GSRS) | |
URL | https://gsrs.ncats.nih.gov/ginas/app/beta/substances/5B0JZH6AB6 | |
Description | The FDA Global Substance Registration System (GSRS) enables the efficient and accurate exchange of information on what substances are in regulated products. Instead of relying on names, which vary across regulatory domains, countries, and regions, the GSRS knowledge base makes it possible for substances to be defined by standardized, scientific descriptions. | |
Explanation | Unless otherwise noted, the contents of the FDA website (www.fda.gov), both text and graphics, are not copyrighted. They are in the public domain and may be republished, reprinted and otherwise used freely by anyone without the need to obtain permission from FDA. Credit to the U.S. Food and Drug Administration as the source is appreciated but not required. | |
Retrosynthesis Analysis
AI-Powered Synthesis Planning: Our tool employs the Template_relevance Pistachio, Template_relevance Bkms_metabolic, Template_relevance Pistachio_ringbreaker, Template_relevance Reaxys, Template_relevance Reaxys_biocatalysis model, leveraging a vast database of chemical reactions to predict feasible synthetic routes.
One-Step Synthesis Focus: Specifically designed for one-step synthesis, it provides concise and direct routes for your target compounds, streamlining the synthesis process.
Accurate Predictions: Utilizing the extensive PISTACHIO, BKMS_METABOLIC, PISTACHIO_RINGBREAKER, REAXYS, REAXYS_BIOCATALYSIS database, our tool offers high-accuracy predictions, reflecting the latest in chemical research and data.
Strategy Settings
Precursor scoring | Relevance Heuristic |
---|---|
Min. plausibility | 0.01 |
Model | Template_relevance |
Template Set | Pistachio/Bkms_metabolic/Pistachio_ringbreaker/Reaxys/Reaxys_biocatalysis |
Top-N result to add to graph | 6 |
Feasible Synthetic Routes
Q1: What is the role of CYP2C9 in the metabolism of desogestrel?
A1: CYP2C9 is a key enzyme responsible for the initial hydroxylation of desogestrel, converting it to 3α-Hydroxydesogestrel. [] This is a crucial step in the bioactivation of desogestrel, as it requires conversion to 3-ketodesogestrel for its progestogenic activity. [] Studies using human liver microsomes and cDNA-expressed CYP2C9 demonstrated the formation of 3α-Hydroxydesogestrel. [] Furthermore, sulfaphenazole, a potent CYP2C9 inhibitor, significantly inhibited this conversion. []
Q2: Are there other enzymes involved in desogestrel metabolism besides CYP2C9?
A2: While CYP2C9 plays a major role, the research suggests CYP2C19 might also contribute to the formation of 3α-Hydroxydesogestrel. [] Combining sulfaphenazole with S-mephenytoin, a CYP2C19 inhibitor, further decreased 3α-Hydroxydesogestrel production in human liver microsomes. [] Inhibitory antibody studies supported this finding, indicating the involvement of both CYP2C9 and CYP2C19. []
Disclaimer and Information on In-Vitro Research Products
Please be aware that all articles and product information presented on BenchChem are intended solely for informational purposes. The products available for purchase on BenchChem are specifically designed for in-vitro studies, which are conducted outside of living organisms. In-vitro studies, derived from the Latin term "in glass," involve experiments performed in controlled laboratory settings using cells or tissues. It is important to note that these products are not categorized as medicines or drugs, and they have not received approval from the FDA for the prevention, treatment, or cure of any medical condition, ailment, or disease. We must emphasize that any form of bodily introduction of these products into humans or animals is strictly prohibited by law. It is essential to adhere to these guidelines to ensure compliance with legal and ethical standards in research and experimentation.