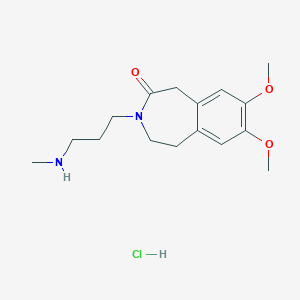
Ivabradine Impurity 12
Overview
Description
Ivabradine Impurity 12 is a degradation product or synthetic byproduct formed during the manufacturing or storage of ivabradine hydrochloride, a selective hyperpolarization-activated cyclic nucleotide-gated (HCN) channel inhibitor used to treat angina pectoris and heart failure. Analytical methods such as high-performance liquid chromatography (HPLC) with gradient elution are critical for separating these impurities, with validation parameters meeting International Council for Harmonisation (ICH) guidelines (RSD ≤10% for impurities, recovery within ±10%) .
Biological Activity
Ivabradine is a medication primarily used to treat certain heart conditions by reducing heart rate without significantly affecting contractility. It acts on the hyperpolarization-activated cyclic nucleotide-gated (HCN) channels, specifically blocking the sodium and potassium ion flow, which leads to a decrease in the pacemaker current of the sinoatrial node. However, during its synthesis, various impurities, including Ivabradine Impurity 12, can be produced. Understanding the biological activity of these impurities is crucial for ensuring drug safety and efficacy.
Chemical Characteristics of this compound
This compound is identified as a degradation product and impurity that arises during the synthesis of Ivabradine. Its structural characteristics can influence its biological activity. The impurity's chemical structure includes specific functional groups that may alter its pharmacokinetic and pharmacodynamic properties compared to the parent compound.
Biological Activity
The biological activity of this compound has been assessed through various studies focusing on its interaction with HCN channels and its overall pharmacological profile.
- Ion Channel Interaction : Similar to Ivabradine, Impurity 12 may interact with HCN channels, although its binding affinity and efficacy could differ significantly. Studies suggest that impurities can alter the pharmacological effects of the main compound by competing for binding sites or modifying channel kinetics .
- Impact on Heart Rate : Preliminary data indicate that this impurity may still exhibit some chronotropic effects; however, these effects are less pronounced than those of Ivabradine itself .
Pharmacokinetics
The pharmacokinetic profile of this compound is critical for understanding its biological activity:
- Absorption : The impurity's absorption characteristics may vary based on its solubility and stability under physiological conditions.
- Metabolism : As an impurity, it is likely metabolized differently than the parent compound. Studies indicate that impurities often undergo rapid metabolism, potentially leading to inactive metabolites .
- Excretion : The elimination pathways for Impurity 12 remain less understood but are expected to involve renal clearance similar to other ivabradine metabolites.
Toxicological Profile
Toxicological assessments have highlighted potential risks associated with impurities:
- Genotoxicity : Forced degradation studies have indicated that some ivabradine degradation products may exhibit genotoxic properties. The Ames test results for this compound suggest a need for further investigation into its mutagenic potential .
- Safety Thresholds : Regulatory guidelines recommend stringent limits on impurity levels in pharmaceutical formulations to mitigate any adverse effects resulting from their biological activity .
Case Studies
Several case studies have documented the implications of impurities in clinical settings:
- A case study involving patients treated with ivabradine highlighted instances where adverse reactions correlated with higher levels of impurities in the formulation. These findings underscore the importance of monitoring impurity levels in therapeutic regimens .
Research Findings
Recent research has provided insights into the biological activity of this compound:
- HPLC Analysis : Advanced analytical techniques such as high-performance liquid chromatography (HPLC) have been employed to quantify impurity levels in ivabradine formulations, revealing significant variability across different batches .
- In Silico Studies : Computational models predict that this compound could have altered binding affinities compared to Ivabradine, suggesting a potential impact on therapeutic outcomes .
Scientific Research Applications
Quality Control in Pharmaceutical Development
Impurity Monitoring
The monitoring of impurities like Ivabradine Impurity 12 is crucial in pharmaceutical development to ensure the safety and effectiveness of the final product. Forced degradation studies have shown that Ivabradine can degrade under various conditions (e.g., exposure to light, heat, and oxidative agents), leading to the formation of several degradation products, including impurities . These studies utilize High-Performance Liquid Chromatography (HPLC) coupled with mass spectrometry to identify and quantify these impurities, which is essential for regulatory compliance and product stability.
Stability Testing
Stability testing of Ivabradine formulations has indicated that certain impurities increase in concentration over time, especially under elevated temperatures. For instance, this compound has been noted for its thermal sensitivity, which raises concerns regarding its presence in drug formulations stored above recommended temperatures . This necessitates rigorous quality control measures to monitor impurity levels throughout the product's shelf life.
Pharmacological Research
Toxicological Assessments
Research has highlighted the importance of assessing the toxicological profiles of impurities like this compound. In silico models have been employed to predict the toxicity of degradation products, including this compound. These assessments often involve Ames tests and evaluations of cytochrome activity to determine potential genotoxic effects . Understanding these toxicological aspects is vital for ensuring patient safety.
Pharmacological Activity
Some degradation products formed during the degradation of Ivabradine, including those related to this compound, have been investigated for their pharmacological activities. Studies suggest that certain degradation products may retain biological activity, which could influence therapeutic outcomes . This aspect is critical for understanding how impurities might affect the overall pharmacodynamics and pharmacokinetics of Ivabradine.
The presence of impurities like this compound poses regulatory challenges for pharmaceutical companies. Regulatory agencies require comprehensive data on impurity profiles as part of the drug approval process. This includes detailed reports on the methods used for impurity identification and quantification, stability data under various conditions, and toxicological assessments .
Q & A
Basic Research Questions
Q. What analytical techniques are recommended for identifying and characterizing Ivabradine Impurity 12?
To characterize this compound, researchers should employ a combination of chromatographic and spectroscopic methods. High-performance liquid chromatography (HPLC) with UV detection is critical for initial separation and quantification, while mass spectrometry (MS) and nuclear magnetic resonance (HNMR) provide structural elucidation . For example, impurities with tautomeric or isomeric properties (e.g., keto-enol tautomers or diastereoisomers) require optimized chromatographic conditions, such as mobile phase adjustments with acetonitrile/methanol ratios and pH modulation to resolve closely eluting peaks .
Q. How do regulatory guidelines (e.g., ICH) influence the acceptable limits of this compound in drug formulations?
The ICH Q6A guideline emphasizes establishing impurity limits based on developmental data and toxicological risk assessment. For this compound, acceptance criteria should account for its molecular structure (C₁₇H₂₆N₂O₃, MW 306.4) and potential toxicity. Decision Tree #1 in ICH Q6A advises extrapolating limits from batch consistency data, though initial filings may require broader acceptance ranges until sufficient process validation is achieved .
Q. What are the stability-indicating parameters for this compound under stressed degradation conditions?
Stress testing (e.g., acid/base hydrolysis, oxidation, thermal degradation) should be conducted to assess Impurity 12’s stability. RP-HPLC methods optimized via chemometric tools (e.g., Box-Behnken Design) can monitor degradation products. Critical parameters include mobile phase composition (e.g., 28 mM phosphate buffer pH 6.0 with acetonitrile/methanol) and column temperature (34°C) to resolve degradation byproducts from the parent compound .
Advanced Research Questions
Q. How can chemometric tools resolve co-elution challenges between this compound and structurally similar impurities?
Principal Component Analysis (PCA) and Box-Behnken Design (BBD) are critical for optimizing chromatographic separations. PCA evaluates column selectivity and pH effects on resolution, while BBD models interactions between variables (e.g., buffer molarity, organic solvent content). For instance, PCA revealed that 70.8% of variation in impurity separation was explained by two principal components, guiding column selection (e.g., Zorbax Eclipse Plus C18) . Derringer’s desirability function further refines multicriteria responses, such as resolving critical peak pairs (e.g., Impurity III/V/VI) by adjusting methanol:acetonitrile ratios (59:41 v/v) .
Q. What experimental strategies address contradictions in impurity quantification data across different analytical batches?
Contradictions often arise from column aging, mobile phase variability, or detector drift. To mitigate this:
- Standardize column conditioning protocols (e.g., equilibration with ≥20 column volumes).
- Use internal standards (e.g., stable isotopically labeled analogs) for MS-based quantification.
- Validate method robustness via fractional factorial designs, testing variables like pH (±0.2 units) and flow rate (±0.1 mL/min) .
Q. How can researchers differentiate this compound from positional isomers (e.g., Impurities III, V, VI) using advanced chromatographic techniques?
Positional isomers require high-resolution chromatography with selectivity adjustments. For example:
- Mobile phase additives : Trimethylamine or heptafluorobutyric acid can enhance selectivity for polar isomers.
- Column chemistry : Charged surface hybrid (CSH) columns improve retention of ionizable impurities.
- Temperature gradients : Elevated temperatures (e.g., 40–50°C) reduce viscosity, improving peak symmetry for co-eluting isomers .
Q. What role does tautomerism play in the analytical profiling of this compound, and how is this addressed methodologically?
Keto-enol tautomerism (e.g., Impurity VII in evidence) can lead to dual peaks under specific conditions. To stabilize tautomers:
- Adjust mobile phase pH to favor one form (e.g., pH 3.0–5.0 for keto-enol equilibrium shifts).
- Use low-temperature chromatography to slow interconversion rates.
- Confirm tautomeric identity via tandem MS/MS fragmentation patterns .
Q. Methodological and Data Analysis Considerations
Q. How should researchers design experiments to validate the specificity of analytical methods for this compound?
A three-tiered approach is recommended:
Forced degradation : Expose Ivabradine to oxidative (H₂O₂), hydrolytic (0.1M HCl/NaOH), and photolytic stress.
Peak purity analysis : Use diode array detectors (DAD) to confirm homogeneity of Impurity 12 peaks.
Spiked recovery studies : Introduce known concentrations of Impurity 12 into placebo matrices to assess interference .
Q. What statistical approaches are suitable for analyzing batch-to-batch variability in Impurity 12 levels?
Multivariate analysis (e.g., ANOVA with Tukey’s post-hoc test) identifies significant variability sources. Control charts (e.g., Shewhart charts) monitor process stability, while Monte Carlo simulations predict impurity distributions under scaled manufacturing conditions .
Q. How can conflicting data on Impurity 12’s pharmacokinetic impact be reconciled in preclinical studies?
Contradictions may arise from species-specific metabolism or dosing regimens. To resolve this:
Comparison with Similar Compounds
Key Analytical Data for Ivabradine Impurities :
Parameter | Ivabradine | Impurities (e.g., X, VII) |
---|---|---|
RSD (precision) | ≤2% | ≤10% |
Recovery (accuracy) | ±2% | ±10% |
Maximum Allowable Concentration (MAC) | N/A | 0.2–0.6% (specification-dependent) |
In one study, Impurity X was detected at 0.1% in tablet formulations, below its MAC of 0.2% .
Comparison with Structurally Similar Impurities
Impurity VII vs. Impurity X
- Structural Features: Impurity VII: Exhibits keto-enol tautomerism, separable via gradient elution . Impurity X: Forms diastereoisomers that cannot be resolved under the same chromatographic conditions .
- Analytical Challenges :
- Impurity VII requires gradient elution for separation, while Impurity X’s diastereoisomers necessitate advanced chiral columns (e.g., cellulose tris-(3-chloro-4-methylphenyl carbamate)) for baseline resolution .
- Detection limits for impurities in S-ivabradine samples can reach 0.05% using validated methods .
Comparison with Pharmacologically Related Compounds
Ivabradine vs. ZD7288
Both compounds reduce heart rate but differ in isoform specificity and therapeutic applicability .
Ivabradine vs. β-Blockers (e.g., Atenolol)
Ivabradine’s heart rate reduction is heart rate-dependent, maximizing efficacy in patients with baseline HR ≥70 bpm .
Data Tables: Key Pharmacodynamic and Clinical Comparisons
Table 1: Anti-Anginal Efficacy in Clinical Trials
Study | Ivabradine (7.5 mg bid) | Atenolol (100 mg od) | Placebo |
---|---|---|---|
TED Improvement (s) | 86.8 ±129.0 | 78.8 ±133.4 | N/A |
Angina Attack Reduction | 59.4% | 66% | 27% (vs. baseline) |
NYHA Class Improvement | Significant (p<0.001) | Moderate (p=0.002) | N/A |
Sources:
Preparation Methods
Synthetic Routes to Ivabradine Impurity 12
Pathway Involving Intermediate Coupling and Hydrogenation
The formation of this compound is closely tied to side reactions during the synthesis of ivabradine. A routine synthesis of ivabradine begins with 7,8-dimethoxy-1,3-dihydrobenzo(d)azepin-2-one (I), which undergoes alkylation with dimethylformamide to form 7,8-dimethoxy-3-(3-chloropropyl)-1,3-dihydro-2H-3-benzazepin-2-one (II) . Subsequent iodination yields intermediate III, which couples with (1S)-4,5-dimethoxy-1-[(methylamino)methyl]benzocyclobutane hydrochloride. Selective hydrogenation then produces ivabradine .
This compound arises when over-alkylation or incomplete hydrogenation occurs at the 3-chloropropyl or 3-iodopropyl intermediates. For instance, residual chloropropyl groups may react with methylamine derivatives under basic conditions, leading to the undesired tertiary amine structure characteristic of Impurity 12 .
Dedicated Synthesis via Multi-Step Organic Reactions
A targeted synthesis of this compound is detailed in patent CN105669554A , which outlines a five-step process:
Step A: Formation of Intermediate III
Intermediate III is synthesized by reacting starting material II with oxalic acid under weak base catalysis (e.g., dicyclohexylcarbodiimide) in polar aprotic solvents like acetonitrile or dichloromethane .
Step B: Acylation to Generate Intermediate V
Intermediate V is produced by acylating starting material IV with N-ethyl-3-halopropylamine (halogen = Cl, Br) in dichloromethane or ethyl acetate .
Step C: Carbonyl Reduction to Intermediate VI
Intermediate V undergoes reduction using sodium borohydride in tetrahydrofuran, yielding intermediate VI with a 94% yield .
Step D: Coupling Reaction to Intermediate VII
Intermediate III reacts with intermediate VI under base catalysis (potassium tert-butoxide or triethylamine) in dimethylformamide or 1,2-dimethoxyethane. This step achieves an 82–85% yield of intermediate VII .
Step E: Hydrochloride Salt Formation
Intermediate VII is treated with hydrogen chloride-saturated isopropanol in acetonitrile, ether, or ethyl acetate, crystallizing this compound with a purity exceeding 99.7% .
Table 1: Optimization of Reaction Conditions for this compound
Step | Solvent | Base/Catalyst | Yield (%) | Purity (%) |
---|---|---|---|---|
A | Acetonitrile | Dicyclohexylcarbodiimide | 92 | 98.5 |
B | Dichloromethane | – | 89 | 97.8 |
C | Tetrahydrofuran | NaBH₄ | 94 | 99.2 |
D | Dimethylformamide | KOtBu | 85 | 99.1 |
E | Acetonitrile | HCl (g) | 75 | 99.75 |
Critical Process Parameters Influencing Impurity Formation
Temperature and Reaction Time
The thermal sensitivity of this compound is well-documented. Stability studies show that its content increases by 0.05% after six months at 30°C, compared to no detectable increase at 25°C . This underscores the need for strict temperature control during synthesis and storage.
Solvent Selection
Polar aprotic solvents like dimethylformamide favor high yields in coupling reactions (Step D), while tetrahydrofuran optimizes carbonyl reduction (Step C) . Ethyl acetate and acetonitrile are preferred for final hydrochloride salt formation due to their low residual solvent risks .
Catalytic Systems
The use of potassium tert-butoxide in Step D enhances reaction efficiency compared to weaker bases like triethylamine, reducing side-product formation .
Analytical Characterization
Spectroscopic Identification
-
¹H NMR (400 MHz, DMSO-d₆) : δ 6.85 (s, 1H, aromatic), 3.85 (s, 3H, OCH₃), 3.20 (t, 2H, NCH₂), 2.70 (s, 3H, NCH₃) .
-
XRPD : Peaks at 8.3°, 11.5°, and 16.7° two-theta confirm crystalline structure .
Chromatographic Purity Assessment
Reverse-phase HPLC with a C18 column (acetonitrile/0.05M TBAH, pH 6.8) resolves Impurity 12 at 12.4 minutes (λ = 272 nm) . Method validation shows a linear response (R² > 0.999) over 0.1–1.0 mg/mL .
Mitigation Strategies in Industrial Synthesis
Process Optimization
-
Reduction of Over-Alkylation : Limiting reaction time and temperature during alkylation steps minimizes tertiary amine formation .
-
Enhanced Purification : Crystallization from ethyl acetate removes residual intermediates, achieving >99.5% purity .
Stability-Indicating Methods
Forced degradation studies (acidic, oxidative, thermal) confirm that HPLC methods reliably quantify Impurity 12 without interference from degradation products .
Regulatory and Quality Considerations
As a "specified impurity" per ICH Q3A guidelines, this compound must be controlled below 0.15% in drug substances . Its genotoxic potential, though unconfirmed, necessitates stringent monitoring during manufacturing .
Properties
IUPAC Name |
7,8-dimethoxy-3-[3-(methylamino)propyl]-2,5-dihydro-1H-3-benzazepin-4-one;hydrochloride | |
---|---|---|
Source | PubChem | |
URL | https://pubchem.ncbi.nlm.nih.gov | |
Description | Data deposited in or computed by PubChem | |
InChI |
InChI=1S/C16H24N2O3.ClH/c1-17-6-4-7-18-8-5-12-9-14(20-2)15(21-3)10-13(12)11-16(18)19;/h9-10,17H,4-8,11H2,1-3H3;1H | |
Source | PubChem | |
URL | https://pubchem.ncbi.nlm.nih.gov | |
Description | Data deposited in or computed by PubChem | |
InChI Key |
AMUXOOATRHJXHN-UHFFFAOYSA-N | |
Source | PubChem | |
URL | https://pubchem.ncbi.nlm.nih.gov | |
Description | Data deposited in or computed by PubChem | |
Canonical SMILES |
CNCCCN1CCC2=CC(=C(C=C2CC1=O)OC)OC.Cl | |
Source | PubChem | |
URL | https://pubchem.ncbi.nlm.nih.gov | |
Description | Data deposited in or computed by PubChem | |
Molecular Formula |
C16H25ClN2O3 | |
Source | PubChem | |
URL | https://pubchem.ncbi.nlm.nih.gov | |
Description | Data deposited in or computed by PubChem | |
Molecular Weight |
328.83 g/mol | |
Source | PubChem | |
URL | https://pubchem.ncbi.nlm.nih.gov | |
Description | Data deposited in or computed by PubChem | |
CAS No. |
85175-52-6 | |
Record name | 7,8-Dimethoxy-3-1,3,4,5-tetrahydro-2H-3-benzazepin-2-one hydrochloride | |
Source | ChemIDplus | |
URL | https://pubchem.ncbi.nlm.nih.gov/substance/?source=chemidplus&sourceid=0085175526 | |
Description | ChemIDplus is a free, web search system that provides access to the structure and nomenclature authority files used for the identification of chemical substances cited in National Library of Medicine (NLM) databases, including the TOXNET system. | |
Record name | 7,8-DIMETHOXY-3-1,3,4,5-TETRAHYDRO-2H-3-BENZAZEPIN-2-ONE HYDROCHLORIDE | |
Source | FDA Global Substance Registration System (GSRS) | |
URL | https://gsrs.ncats.nih.gov/ginas/app/beta/substances/D65SP8A4F6 | |
Description | The FDA Global Substance Registration System (GSRS) enables the efficient and accurate exchange of information on what substances are in regulated products. Instead of relying on names, which vary across regulatory domains, countries, and regions, the GSRS knowledge base makes it possible for substances to be defined by standardized, scientific descriptions. | |
Explanation | Unless otherwise noted, the contents of the FDA website (www.fda.gov), both text and graphics, are not copyrighted. They are in the public domain and may be republished, reprinted and otherwise used freely by anyone without the need to obtain permission from FDA. Credit to the U.S. Food and Drug Administration as the source is appreciated but not required. | |
Synthesis routes and methods
Procedure details
Retrosynthesis Analysis
AI-Powered Synthesis Planning: Our tool employs the Template_relevance Pistachio, Template_relevance Bkms_metabolic, Template_relevance Pistachio_ringbreaker, Template_relevance Reaxys, Template_relevance Reaxys_biocatalysis model, leveraging a vast database of chemical reactions to predict feasible synthetic routes.
One-Step Synthesis Focus: Specifically designed for one-step synthesis, it provides concise and direct routes for your target compounds, streamlining the synthesis process.
Accurate Predictions: Utilizing the extensive PISTACHIO, BKMS_METABOLIC, PISTACHIO_RINGBREAKER, REAXYS, REAXYS_BIOCATALYSIS database, our tool offers high-accuracy predictions, reflecting the latest in chemical research and data.
Strategy Settings
Precursor scoring | Relevance Heuristic |
---|---|
Min. plausibility | 0.01 |
Model | Template_relevance |
Template Set | Pistachio/Bkms_metabolic/Pistachio_ringbreaker/Reaxys/Reaxys_biocatalysis |
Top-N result to add to graph | 6 |
Feasible Synthetic Routes
Disclaimer and Information on In-Vitro Research Products
Please be aware that all articles and product information presented on BenchChem are intended solely for informational purposes. The products available for purchase on BenchChem are specifically designed for in-vitro studies, which are conducted outside of living organisms. In-vitro studies, derived from the Latin term "in glass," involve experiments performed in controlled laboratory settings using cells or tissues. It is important to note that these products are not categorized as medicines or drugs, and they have not received approval from the FDA for the prevention, treatment, or cure of any medical condition, ailment, or disease. We must emphasize that any form of bodily introduction of these products into humans or animals is strictly prohibited by law. It is essential to adhere to these guidelines to ensure compliance with legal and ethical standards in research and experimentation.