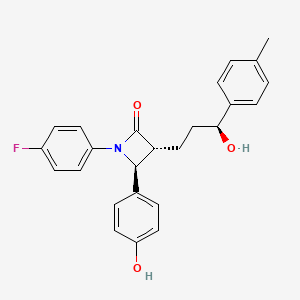
(3R,4S)-1-(4-Fluorophenyl)-3-((S)-3-hydroxy-3-(P-tolyl)propyl)-4-(4-hydroxyphenyl)azetidin-2-one
Overview
Description
An impurity of Ezetimibe. Ezetimibe inhibits intestinal cholesterol absorption by preventing cholesterol uptake by the Niemann-Pick C1-like 1 (NPC1L30) protein, a cholesterol transporter located in the apical membrane of enterocytes.
Scientific Research Applications
Crystal Structure Analysis
(3R,4S)-1-(4-Fluorophenyl)-3-((S)-3-hydroxy-3-(P-tolyl)propyl)-4-(4-hydroxyphenyl)azetidin-2-one, also known as Ezetimibe, has been studied for its crystal structure. The compound crystallizes in the space group P2(1)2(1)2(1), with molecules forming an extended hydrogen-bond architecture, aiding in understanding its chemical interactions and stability (Brüning, Alig, & Schmidt, 2010).
Microbial Reduction and Synthesis
Research includes the microbial reduction of Ezetimibe using the whole cell catalyst Rhodococcus fascians MO22. This process facilitates the conversion of a ketone intermediate into Ezetimibe, optimizing the synthesis route (Kyslíková et al., 2010).
Fungal Biotransformation
Fungi like Beauvaria bassiana and Cunninghamella blakesleeana have been used to metabolize Ezetimibe, demonstrating their capacity to process halogenated compounds and drugs targeted by the UDP-Glucuronyl Transferase System. This biotransformation leads to the creation of various metabolites, showing potential for drug modification and waste processing (Pervaiz et al., 2014).
Synthesis of Derivatives and Biological Activity
The synthesis of novel phenyl pyrazoline derivatives from 4-(4-hydroxyphenyl)-3-chloro-1-{4-(5-(substituted phenyl)-1-phenyl-4,5-dihydro-pyrazol-3-yl)phenyl}azetidin-2-one and their antimicrobial activity has been researched. This opens avenues for developing new pharmaceutical compounds with potential medical applications (Shah & Patel, 2012).
Metabolic Pathways and Glucuronidation
Understanding the metabolic pathways, particularly the glucuronidation of Ezetimibe, has been a subject of study. Identifying the specific human liver and intestinal UDP-glucuronosyltransferase enzymes involved in this process is crucial for appreciating how the body processes and metabolizes the compound (Ghosal et al., 2004).
Cholesterol Absorption Inhibition
Studies on Ezetimibe have shown its effectiveness in inhibiting intestinal cholesterol absorption, thereby impacting plasma cholesterol levels. This property makes it significant in the context of treating hypercholesterolemia (van Heek, Compton, & Davis, 2001).
properties
IUPAC Name |
(3R,4S)-1-(4-fluorophenyl)-3-[(3S)-3-hydroxy-3-(4-methylphenyl)propyl]-4-(4-hydroxyphenyl)azetidin-2-one | |
---|---|---|
Source | PubChem | |
URL | https://pubchem.ncbi.nlm.nih.gov | |
Description | Data deposited in or computed by PubChem | |
InChI |
InChI=1S/C25H24FNO3/c1-16-2-4-17(5-3-16)23(29)15-14-22-24(18-6-12-21(28)13-7-18)27(25(22)30)20-10-8-19(26)9-11-20/h2-13,22-24,28-29H,14-15H2,1H3/t22-,23+,24-/m1/s1 | |
Source | PubChem | |
URL | https://pubchem.ncbi.nlm.nih.gov | |
Description | Data deposited in or computed by PubChem | |
InChI Key |
ULDWXJWBGUZASZ-TZRRMPRUSA-N | |
Source | PubChem | |
URL | https://pubchem.ncbi.nlm.nih.gov | |
Description | Data deposited in or computed by PubChem | |
Canonical SMILES |
CC1=CC=C(C=C1)C(CCC2C(N(C2=O)C3=CC=C(C=C3)F)C4=CC=C(C=C4)O)O | |
Source | PubChem | |
URL | https://pubchem.ncbi.nlm.nih.gov | |
Description | Data deposited in or computed by PubChem | |
Isomeric SMILES |
CC1=CC=C(C=C1)[C@H](CC[C@@H]2[C@H](N(C2=O)C3=CC=C(C=C3)F)C4=CC=C(C=C4)O)O | |
Source | PubChem | |
URL | https://pubchem.ncbi.nlm.nih.gov | |
Description | Data deposited in or computed by PubChem | |
Molecular Formula |
C25H24FNO3 | |
Source | PubChem | |
URL | https://pubchem.ncbi.nlm.nih.gov | |
Description | Data deposited in or computed by PubChem | |
Molecular Weight |
405.5 g/mol | |
Source | PubChem | |
URL | https://pubchem.ncbi.nlm.nih.gov | |
Description | Data deposited in or computed by PubChem | |
Retrosynthesis Analysis
AI-Powered Synthesis Planning: Our tool employs the Template_relevance Pistachio, Template_relevance Bkms_metabolic, Template_relevance Pistachio_ringbreaker, Template_relevance Reaxys, Template_relevance Reaxys_biocatalysis model, leveraging a vast database of chemical reactions to predict feasible synthetic routes.
One-Step Synthesis Focus: Specifically designed for one-step synthesis, it provides concise and direct routes for your target compounds, streamlining the synthesis process.
Accurate Predictions: Utilizing the extensive PISTACHIO, BKMS_METABOLIC, PISTACHIO_RINGBREAKER, REAXYS, REAXYS_BIOCATALYSIS database, our tool offers high-accuracy predictions, reflecting the latest in chemical research and data.
Strategy Settings
Precursor scoring | Relevance Heuristic |
---|---|
Min. plausibility | 0.01 |
Model | Template_relevance |
Template Set | Pistachio/Bkms_metabolic/Pistachio_ringbreaker/Reaxys/Reaxys_biocatalysis |
Top-N result to add to graph | 6 |
Feasible Synthetic Routes
Disclaimer and Information on In-Vitro Research Products
Please be aware that all articles and product information presented on BenchChem are intended solely for informational purposes. The products available for purchase on BenchChem are specifically designed for in-vitro studies, which are conducted outside of living organisms. In-vitro studies, derived from the Latin term "in glass," involve experiments performed in controlled laboratory settings using cells or tissues. It is important to note that these products are not categorized as medicines or drugs, and they have not received approval from the FDA for the prevention, treatment, or cure of any medical condition, ailment, or disease. We must emphasize that any form of bodily introduction of these products into humans or animals is strictly prohibited by law. It is essential to adhere to these guidelines to ensure compliance with legal and ethical standards in research and experimentation.