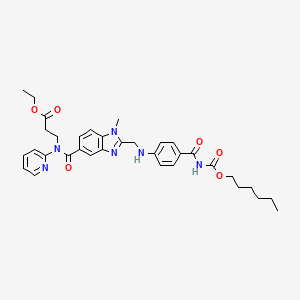
Dabigatran Impurity 8
Overview
Description
For instance, impurities such as Impurity 9 (C₁₉H₂₂ClN₅O₂, MW: 387.87) and Impurity 37 (C₂₄H₂₉N₅O₄, MW: 451.52) are well-characterized by their molecular structures, formation pathways, and detection methods . Impurity 8 is hypothesized to belong to the same class of carbamate or benzimidazole-derived byproducts, formed via incomplete ester hydrolysis or oxidative degradation during manufacturing . Regulatory guidelines (ICH Q3A) mandate strict control of such impurities, with identification thresholds ≥0.1% and qualification thresholds ≥0.3% .
Preparation Methods
Synthetic Routes and Key Reaction Mechanisms
Amidation via Carbodiimide-Mediated Coupling
A primary route involves the reaction of intermediate SM02 (ethyl 3-((3-amino-4-(methylamino)benzoyl)(pyridin-2-yl)amino)propanoate) with 1,1'-carbonyldiimidazole (CDI) in aprotic solvents.
-
Reaction Conditions :
This step generates an activated carbonyl intermediate, which undergoes intramolecular cyclization to form the benzimidazole core. Side reactions, such as incomplete cyclization or over-reaction, yield Impurity 8 .
Hydrolysis and Recrystallization
Controlled hydrolysis of Dabigatran Etexilate under alkaline conditions produces Impurity 8 as a degradant:
-
Conditions :
-
Work-Up : Acidification to pH 3.5 followed by ethyl acetate recrystallization yields 37% purity .
Critical Process Parameters and Optimization
Solvent Selection
Solvent polarity directly impacts impurity formation:
Polar aprotic solvents (e.g., DCM) enhance reaction homogeneity, while toluene minimizes side products via azeotropic distillation .
Temperature and Reaction Time
Purification and Isolation Strategies
Recrystallization
-
Ethyl Acetate Pulping :
Preparative Chromatography
Analytical Characterization
LC-MS/MS Profiling
-
Fragmentation Pattern :
NMR Spectroscopy
Industrial-Scale Challenges and Solutions
Byproduct Minimization
Regulatory Compliance
-
Stability Studies : Impurity 8 increases by 0.02% per month under accelerated conditions (40°C/75% RH) .
Comparative Data on Synthesis Methods
Chemical Reactions Analysis
Types of Reactions
Dabigatran Impurity 8 undergoes various chemical reactions, including:
Oxidation: This reaction involves the addition of oxygen or the removal of hydrogen.
Reduction: This reaction involves the addition of hydrogen or the removal of oxygen.
Substitution: This reaction involves the replacement of one functional group with another.
Common Reagents and Conditions
Common reagents used in these reactions include oxidizing agents like hydrogen peroxide, reducing agents like sodium borohydride, and various nucleophiles for substitution reactions . The reaction conditions typically involve controlled temperatures and pH levels to ensure the desired outcome .
Major Products Formed
The major products formed from these reactions depend on the specific reagents and conditions used. For example, oxidation may lead to the formation of nitroso derivatives, while reduction may yield amine derivatives .
Scientific Research Applications
Analytical Method Development
Dabigatran Impurity 8 is primarily studied in the context of developing and validating analytical methods for detecting and quantifying impurities in pharmaceutical formulations. Several studies have focused on high-performance liquid chromatography (HPLC) and liquid chromatography-mass spectrometry (LC-MS) techniques to achieve this goal.
Case Study: LC-MS Method Validation
A study by Arous and Al-Mardini developed a sensitive LC-MS method for analyzing dabigatran etexilate and its related impurities, including this compound. The method demonstrated high specificity and sensitivity, with limits of detection (LOD) and quantification (LOQ) established at acceptable levels. The validation process adhered to the International Council for Harmonisation (ICH) guidelines, confirming the method's robustness for routine analysis in quality control laboratories .
Parameter | Value |
---|---|
LOD (ppm) | 33 - 55 |
LOQ (ppm) | 112 - 168 |
Recovery (%) | 80 - 120 |
RSD (%) | <2 |
This validated method is crucial for ensuring that dabigatran etexilate formulations meet regulatory standards regarding impurity levels.
Quality Control in Pharmaceutical Manufacturing
The presence of impurities like this compound during the synthesis of dabigatran etexilate raises concerns about drug safety and efficacy. Understanding its formation and behavior is essential for pharmaceutical manufacturers to comply with Good Manufacturing Practices (GMP).
Quality Assurance Insights
Research indicates that controlling the synthesis process to minimize impurities is vital. Various synthetic routes have been optimized to reduce the formation of this compound while maximizing yield. The insights gained from these studies inform regulatory guidelines concerning acceptable impurity levels in pharmaceutical products .
While primarily regarded as an impurity, there is ongoing research into the potential biological activity of this compound. Understanding its pharmacological properties can provide insights into its safety profile when present in drug formulations.
Case Study: Pharmacological Evaluation
Recent studies have begun to evaluate the pharmacological effects of dabigatran-related impurities, including this compound. Preliminary findings suggest that impurities may exhibit varying degrees of biological activity, potentially influencing the overall therapeutic effect of dabigatran etexilate .
Regulatory Compliance and Risk Assessment
Regulatory agencies require comprehensive risk assessments concerning impurities in pharmaceuticals. The study of this compound contributes to understanding how such impurities affect patient safety and treatment outcomes.
Risk Management Strategies
The analysis of medication errors associated with dabigatran emphasizes the importance of proper dosing and administration practices to mitigate risks linked to impurities like this compound. Reports indicate that serious medication errors often arise from confusion regarding dosing regimens influenced by the presence of various impurities .
Mechanism of Action
Dabigatran Impurity 8, like Dabigatran, acts as a thrombin inhibitor. It binds to the active site of thrombin, preventing the conversion of fibrinogen to fibrin, thereby inhibiting blood clot formation . The molecular targets include thrombin and other components of the coagulation cascade .
Comparison with Similar Compounds
Structural and Functional Similarities
Dabigatran-related impurities share core structural motifs, such as benzimidazole rings, pyridyl groups, or carbamate linkages, which influence their chemical reactivity and biological activity. Key impurities include:
Key Observations :
- Impurity 9 and Impurity 37 differ in side-chain modifications, affecting solubility and hygroscopicity .
- Impurity A retains the pyridyl-carboxamide pharmacophore but lacks the prodrug’s ester group, reducing thrombin inhibition potency .
Analytical and Regulatory Considerations
Detection Methods :
- HPLC-MS and LC-MS/MS are gold-standard techniques for impurity profiling, offering specificity for structural isomers (e.g., acyl glucuronides of dabigatran) .
- Accelerated stability studies under varied pH, temperature, and humidity conditions are critical for identifying degradation pathways .
Regulatory Thresholds :
- Identification threshold : ≥0.1% of API (Active Pharmaceutical Ingredient).
- Qualification threshold : ≥0.3%, requiring toxicological assessment .
Comparative Data Table: Pharmacological and Analytical Properties
Biological Activity
Dabigatran Impurity 8, a derivative of the anticoagulant dabigatran, has garnered attention due to its potential biological activities and implications in pharmacology. This article explores the biological activity of this compound, including its mechanisms of action, pharmacokinetics, and relevant studies.
Chemical Structure and Properties
This compound is identified by the chemical formula and has a molecular weight of approximately 596.73 g/mol. Its structural characteristics are pivotal in determining its interaction with biological targets, particularly thrombin.
Dabigatran, the active form derived from Dabigatran Etexilate, functions primarily as a direct thrombin inhibitor . It inhibits both free and fibrin-bound thrombin, which is crucial for converting fibrinogen to fibrin during the coagulation cascade. Consequently, this inhibition prevents thrombus formation . While this compound shares some structural similarities with dabigatran, its specific biological activity and effects on coagulation pathways require further investigation.
Biological Activity and Pharmacokinetics
The pharmacokinetic profile of dabigatran suggests that it reaches peak plasma concentrations approximately two hours post-administration, with a terminal elimination half-life ranging from 12 to 14 hours . The bioavailability of dabigatran can be significantly affected by gastric pH levels; for instance, studies indicate that the presence of proton pump inhibitors can reduce dabigatran levels by up to 70% .
Table 1: Pharmacokinetic Parameters of Dabigatran
Parameter | Value |
---|---|
Peak Plasma Concentration (Cmax) | ~30% higher at steady state than after first dose |
Time to Cmax | ~2 hours |
Terminal Half-life | 12-14 hours |
Urinary Excretion | Up to 80% of the dose |
Case Studies and Research Findings
Recent studies have highlighted the importance of understanding dabigatran's impurities in clinical settings. For example, a meta-analysis involving patients undergoing catheter ablation for atrial fibrillation compared dabigatran therapy with traditional warfarin treatment. The findings showed no significant differences in thromboembolic events between the two groups, indicating that dabigatran maintains efficacy comparable to warfarin during procedures .
Table 2: Summary of Clinical Findings on Dabigatran Use
Study Type | Participants | Thromboembolic Events (Warfarin vs. Dabigatran) | Major Bleeding Events (Warfarin vs. Dabigatran) |
---|---|---|---|
Retrospective Observational | 3648 | 3 vs. 9 | Similar rates |
Q & A
Basic Questions
Q. What analytical methodologies are recommended for structural confirmation of Dabigatran Impurity 8?
To confirm the structure of this compound, researchers should employ a combination of spectroscopic techniques (e.g., NMR , LC-MS/MS , HRMS ) and chromatographic methods (e.g., HPLC with UV detection). For novel impurities, comparative analysis against synthetic reference standards is critical. Structural elucidation requires full spectral interpretation, including fragmentation patterns in mass spectrometry and cross-validation with synthetic pathways. Newly synthesized impurities must meet purity thresholds (>95%) and be characterized with batch-specific analytical data .
Q. How should researchers design experiments to quantify this compound in drug substances?
A validated stability-indicating HPLC or UPLC method is essential. Key steps include:
- Forced degradation studies under acidic, alkaline, oxidative, and thermal conditions to assess method specificity.
- Linearity testing across 50–150% of the target concentration (e.g., 0.05–0.15 μg/mL).
- Accuracy and precision validation using spiked samples (e.g., 80%, 100%, 120% recovery).
- Detection/quantitation limits aligned with ICH Q3A guidelines (e.g., ≤0.1% w/w for identified impurities) .
Q. What regulatory standards govern impurity profiling for this compound?
Researchers must adhere to ICH Q3A (R2) for impurity identification thresholds and FDA guidance for method validation. Key requirements include:
- Reporting all impurities ≥0.10% in drug substances.
- Providing structural evidence for specified impurities.
- Validating methods for specificity, linearity, accuracy, and robustness.
- Cross-referencing pharmacopeial standards (e.g., USP) where applicable .
Advanced Research Questions
Q. How can contradictory data on this compound’s toxicity be resolved in preclinical studies?
Contradictions often arise from differences in sample matrices , degradation conditions , or analytical sensitivity . Mitigation strategies include:
- Meta-analysis of existing studies to identify methodological inconsistencies (e.g., LC-MS vs. GC-MS).
- In vitro toxicity assays (e.g., Ames test for genotoxicity) using well-characterized impurity samples.
- Dose-response modeling to establish thresholds for adverse effects, supported by impurity spiking in biological matrices .
Q. What experimental approaches are effective for studying degradation pathways of this compound?
Advanced degradation studies require:
- Stress testing under controlled conditions (e.g., pH, temperature, light) to simulate real-world stability.
- Kinetic modeling to predict degradation rates using Arrhenius equations.
- Isolation of degradation products via preparative chromatography followed by structural elucidation.
- Computational chemistry (e.g., DFT calculations) to predict reactive intermediates .
Q. How can researchers address the lack of reference standards for this compound?
When reference materials are unavailable:
- In-house synthesis with rigorous purity validation (≥95%).
- Cross-laboratory collaboration to share analytical data (e.g., spectral libraries).
- Surrogate markers using structurally analogous compounds for method development.
- Regulatory exemptions under ICH Q6A if justified by risk assessments .
Q. What strategies improve the detection of trace-level this compound in complex matrices?
Enhance sensitivity via:
- Mass spectrometry enhancements : Ion mobility spectrometry (IMS) for matrix interference reduction.
- Derivatization techniques to improve ionization efficiency.
- Multi-dimensional chromatography (e.g., LC-LC or LC-GC) for peak resolution.
- Machine learning algorithms to deconvolute overlapping chromatographic signals .
Q. Data Interpretation and Reporting
Q. How should researchers handle variability in impurity quantification across batches?
- Statistical process control (SPC) : Use control charts (e.g., X-bar and R charts) to monitor batch-to-batch variability.
- Root-cause analysis : Investigate synthesis conditions (e.g., catalyst purity, reaction time) using design of experiments (DoE).
- Outlier assessment : Apply Grubbs’ test to exclude anomalous data points .
Q. What best practices ensure reproducibility in impurity profiling studies?
- Detailed method documentation : Include instrument parameters, column specifications, and mobile phase gradients.
- Open-data sharing : Deposit raw chromatograms and spectral data in repositories like Zenodo or Figshare.
- Inter-laboratory validation : Participate in round-robin testing to verify method robustness .
Tables for Reference
Analytical Parameter | ICH/FDA Requirement | Example for this compound |
---|---|---|
Linearity Range | 50–150% of target concentration | 0.05–0.15 μg/mL |
Accuracy (% Recovery) | 98–102% | 99.2% ± 1.5% (n=6) |
Quantitation Limit | ≤0.10% w/w | 0.03 μg/mL (S/N ≥10) |
Precision (RSD) | ≤2.0% | 1.8% intra-day, 2.1% inter-day |
Key Citations
Properties
IUPAC Name |
ethyl 3-[[2-[[4-(hexoxycarbonylcarbamoyl)anilino]methyl]-1-methylbenzimidazole-5-carbonyl]-pyridin-2-ylamino]propanoate | |
---|---|---|
Source | PubChem | |
URL | https://pubchem.ncbi.nlm.nih.gov | |
Description | Data deposited in or computed by PubChem | |
InChI |
InChI=1S/C34H40N6O6/c1-4-6-7-10-21-46-34(44)38-32(42)24-12-15-26(16-13-24)36-23-30-37-27-22-25(14-17-28(27)39(30)3)33(43)40(20-18-31(41)45-5-2)29-11-8-9-19-35-29/h8-9,11-17,19,22,36H,4-7,10,18,20-21,23H2,1-3H3,(H,38,42,44) | |
Source | PubChem | |
URL | https://pubchem.ncbi.nlm.nih.gov | |
Description | Data deposited in or computed by PubChem | |
InChI Key |
FZKPGDBVPFJTPG-UHFFFAOYSA-N | |
Source | PubChem | |
URL | https://pubchem.ncbi.nlm.nih.gov | |
Description | Data deposited in or computed by PubChem | |
Canonical SMILES |
CCCCCCOC(=O)NC(=O)C1=CC=C(C=C1)NCC2=NC3=C(N2C)C=CC(=C3)C(=O)N(CCC(=O)OCC)C4=CC=CC=N4 | |
Source | PubChem | |
URL | https://pubchem.ncbi.nlm.nih.gov | |
Description | Data deposited in or computed by PubChem | |
Molecular Formula |
C34H40N6O6 | |
Source | PubChem | |
URL | https://pubchem.ncbi.nlm.nih.gov | |
Description | Data deposited in or computed by PubChem | |
Molecular Weight |
628.7 g/mol | |
Source | PubChem | |
URL | https://pubchem.ncbi.nlm.nih.gov | |
Description | Data deposited in or computed by PubChem | |
CAS No. |
1408238-40-3 | |
Record name | Dabigatran impurity 8 | |
Source | ChemIDplus | |
URL | https://pubchem.ncbi.nlm.nih.gov/substance/?source=chemidplus&sourceid=1408238403 | |
Description | ChemIDplus is a free, web search system that provides access to the structure and nomenclature authority files used for the identification of chemical substances cited in National Library of Medicine (NLM) databases, including the TOXNET system. | |
Record name | Ethyl 3-(2-{[(4-{[(hexyloxy)carbonyl]carbamoyl}phenyl)amino]methyl}-1-methyl-N-(pyridin-2-yl)-1H-benzimidazole-5-carboxamido)propanoate | |
Source | FDA Global Substance Registration System (GSRS) | |
URL | https://gsrs.ncats.nih.gov/ginas/app/beta/substances/HW3X7HP2ZR | |
Description | The FDA Global Substance Registration System (GSRS) enables the efficient and accurate exchange of information on what substances are in regulated products. Instead of relying on names, which vary across regulatory domains, countries, and regions, the GSRS knowledge base makes it possible for substances to be defined by standardized, scientific descriptions. | |
Explanation | Unless otherwise noted, the contents of the FDA website (www.fda.gov), both text and graphics, are not copyrighted. They are in the public domain and may be republished, reprinted and otherwise used freely by anyone without the need to obtain permission from FDA. Credit to the U.S. Food and Drug Administration as the source is appreciated but not required. | |
Synthesis routes and methods
Procedure details
Retrosynthesis Analysis
AI-Powered Synthesis Planning: Our tool employs the Template_relevance Pistachio, Template_relevance Bkms_metabolic, Template_relevance Pistachio_ringbreaker, Template_relevance Reaxys, Template_relevance Reaxys_biocatalysis model, leveraging a vast database of chemical reactions to predict feasible synthetic routes.
One-Step Synthesis Focus: Specifically designed for one-step synthesis, it provides concise and direct routes for your target compounds, streamlining the synthesis process.
Accurate Predictions: Utilizing the extensive PISTACHIO, BKMS_METABOLIC, PISTACHIO_RINGBREAKER, REAXYS, REAXYS_BIOCATALYSIS database, our tool offers high-accuracy predictions, reflecting the latest in chemical research and data.
Strategy Settings
Precursor scoring | Relevance Heuristic |
---|---|
Min. plausibility | 0.01 |
Model | Template_relevance |
Template Set | Pistachio/Bkms_metabolic/Pistachio_ringbreaker/Reaxys/Reaxys_biocatalysis |
Top-N result to add to graph | 6 |
Feasible Synthetic Routes
Disclaimer and Information on In-Vitro Research Products
Please be aware that all articles and product information presented on BenchChem are intended solely for informational purposes. The products available for purchase on BenchChem are specifically designed for in-vitro studies, which are conducted outside of living organisms. In-vitro studies, derived from the Latin term "in glass," involve experiments performed in controlled laboratory settings using cells or tissues. It is important to note that these products are not categorized as medicines or drugs, and they have not received approval from the FDA for the prevention, treatment, or cure of any medical condition, ailment, or disease. We must emphasize that any form of bodily introduction of these products into humans or animals is strictly prohibited by law. It is essential to adhere to these guidelines to ensure compliance with legal and ethical standards in research and experimentation.