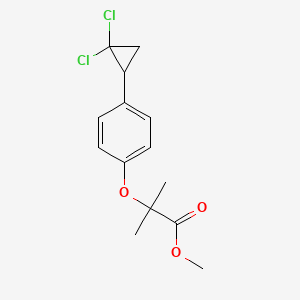
Ciprofibrate Impurity D
Overview
Description
Mechanism of Action
Target of Action
Ciprofibrate Impurity D primarily targets the Peroxisome Proliferator-Activated Receptor alpha (PPARα) . PPARα is a nuclear receptor protein that plays a crucial role in the regulation of lipid metabolism, including the breakdown of fats and cholesterol .
Mode of Action
This compound is believed to function as an agonist of PPARα . As an agonist, it binds to PPARα and activates it, leading to an increase in the receptor’s activity . This activation results in the acceleration of the breakdown of dietary fat, especially triglycerides and cholesterol, thus helping to clear the blood of cholesterol and triglycerides .
Biochemical Pathways
The activation of PPARα by this compound affects several biochemical pathways. It leads to a marked reduction in the levels of very low-density lipoprotein (VLDL)-1 and VLDL-2, which are rich in triglycerides . It also results in a reduction in the levels of low-density lipoprotein (LDL), particularly dense LDL particles . Concurrently, it induces an elevation in the levels of high-density lipoprotein (HDL), particularly HDL-3 .
Action Environment
The action, efficacy, and stability of this compound can be influenced by various environmental factors. For instance, the levels of lipids in the body, which can be affected by diet and lifestyle, can impact the effectiveness of this compound . High levels of lipids can lead to the deposition of excess fat onto the walls of blood vessels, potentially reducing the efficacy of this compound .
Biochemical Analysis
Biochemical Properties
Ciprofibrate Impurity D plays a role in various biochemical reactions. It interacts with several enzymes, proteins, and other biomolecules. For instance, it has been observed to interact with peroxisome proliferator-activated receptor alpha (PPARα), which is a key regulator of lipid metabolism . The nature of these interactions involves the activation of PPARα, leading to changes in the expression of genes involved in lipid metabolism.
Cellular Effects
This compound influences various types of cells and cellular processes. It affects cell function by modulating cell signaling pathways, gene expression, and cellular metabolism. For example, this compound has been shown to stimulate high-density lipoprotein (HDL)-mediated cellular cholesterol efflux, which is crucial for maintaining cellular cholesterol homeostasis . Additionally, it can impact the expression of genes involved in lipid metabolism, thereby influencing cellular lipid levels.
Molecular Mechanism
The molecular mechanism of action of this compound involves its binding interactions with biomolecules, enzyme inhibition or activation, and changes in gene expression. This compound acts by activating PPARα, which in turn regulates the expression of genes involved in lipid metabolism . This activation leads to increased fatty acid oxidation and decreased triglyceride levels in the liver. Additionally, this compound can inhibit the activity of certain enzymes involved in cholesterol biosynthesis, further contributing to its lipid-lowering effects .
Temporal Effects in Laboratory Settings
In laboratory settings, the effects of this compound can change over time. Studies have shown that this compound is relatively stable under standard laboratory conditions . Its long-term effects on cellular function have been observed in both in vitro and in vivo studies. For instance, prolonged exposure to this compound can lead to sustained activation of PPARα and continuous modulation of lipid metabolism .
Dosage Effects in Animal Models
The effects of this compound vary with different dosages in animal models. At lower doses, this compound effectively reduces lipid levels without causing significant adverse effects . At higher doses, it can lead to toxic effects such as hepatotoxicity and myopathy . These threshold effects highlight the importance of careful dosage optimization in therapeutic applications.
Metabolic Pathways
This compound is involved in several metabolic pathways, including those related to lipid metabolism. It interacts with enzymes such as acyl-CoA oxidase and carnitine palmitoyltransferase, which are involved in fatty acid oxidation . Additionally, this compound can affect metabolic flux by altering the levels of key metabolites such as triglycerides and cholesterol .
Transport and Distribution
Within cells and tissues, this compound is transported and distributed through interactions with specific transporters and binding proteins. For example, it can bind to albumin in the bloodstream, facilitating its transport to various tissues . Once inside the cells, this compound can accumulate in lipid droplets and other cellular compartments involved in lipid metabolism .
Subcellular Localization
The subcellular localization of this compound is crucial for its activity and function. It is primarily localized in the cytoplasm, where it interacts with PPARα and other proteins involved in lipid metabolism . Additionally, this compound can undergo post-translational modifications that direct it to specific cellular compartments, such as the endoplasmic reticulum and mitochondria .
Preparation Methods
The synthesis of Ciprofibrate Impurity D involves several steps:
Starting Materials: The synthesis begins with the preparation of 2,2-dichlorocyclopropyl phenol.
Reaction with Methyl 2-bromo-2-methylpropanoate: The 2,2-dichlorocyclopropyl phenol is reacted with methyl 2-bromo-2-methylpropanoate in the presence of a base such as potassium carbonate.
Esterification: The resulting product undergoes esterification to form Methyl 2-[4-[(1RS)-2,2-dichlorocyclopropyl]phenoxy]-2-methylpropanoate.
Industrial production methods typically involve large-scale synthesis using similar reaction conditions but optimized for higher yields and purity.
Chemical Reactions Analysis
Ciprofibrate Impurity D undergoes various chemical reactions:
Oxidation: It can be oxidized using oxidizing agents such as potassium permanganate or chromium trioxide.
Reduction: Reduction reactions can be carried out using reducing agents like lithium aluminum hydride.
Substitution: Nucleophilic substitution reactions can occur, especially at the cyclopropyl ring, using reagents like sodium methoxide.
Hydrolysis: The ester group can be hydrolyzed under acidic or basic conditions to yield the corresponding carboxylic acid.
Major products formed from these reactions include the oxidized, reduced, or hydrolyzed derivatives of this compound.
Scientific Research Applications
Ciprofibrate Impurity D is used in various scientific research applications:
Pharmaceutical Research: It serves as a reference standard for the development and validation of analytical methods, quality control, and stability studies of Ciprofibrate formulations.
Analytical Chemistry: It is used in the identification and quantification of impurities in pharmaceutical products.
Biological Studies: Research involving the metabolism and pharmacokinetics of Ciprofibrate may use this impurity to study its behavior in biological systems.
Comparison with Similar Compounds
Ciprofibrate Impurity D can be compared with other impurities of Ciprofibrate:
Ciprofibrate Impurity A: 2-(4-Ethenylphenoxy)-2-methylpropanoic Acid
Ciprofibrate Impurity B: 4-[(1RS)-2,2-Dichlorocyclopropyl]phenol
Ciprofibrate Impurity C: 2-[4-[(1RS)-2,2-Dichlorocyclopropyl]phenoxy]-2-methylpropan-1-ol
Ciprofibrate Impurity E: Ethyl 2-[4-[(1RS)-2,2-dichlorocyclopropyl]phenoxy]-2-methylpropanoate
This compound is unique due to its specific ester structure, which differentiates it from other impurities in terms of its chemical properties and reactivity.
Properties
IUPAC Name |
methyl 2-[4-(2,2-dichlorocyclopropyl)phenoxy]-2-methylpropanoate | |
---|---|---|
Source | PubChem | |
URL | https://pubchem.ncbi.nlm.nih.gov | |
Description | Data deposited in or computed by PubChem | |
InChI |
InChI=1S/C14H16Cl2O3/c1-13(2,12(17)18-3)19-10-6-4-9(5-7-10)11-8-14(11,15)16/h4-7,11H,8H2,1-3H3 | |
Source | PubChem | |
URL | https://pubchem.ncbi.nlm.nih.gov | |
Description | Data deposited in or computed by PubChem | |
InChI Key |
QKMIJFOKKANKAZ-UHFFFAOYSA-N | |
Source | PubChem | |
URL | https://pubchem.ncbi.nlm.nih.gov | |
Description | Data deposited in or computed by PubChem | |
Canonical SMILES |
CC(C)(C(=O)OC)OC1=CC=C(C=C1)C2CC2(Cl)Cl | |
Source | PubChem | |
URL | https://pubchem.ncbi.nlm.nih.gov | |
Description | Data deposited in or computed by PubChem | |
Molecular Formula |
C14H16Cl2O3 | |
Source | PubChem | |
URL | https://pubchem.ncbi.nlm.nih.gov | |
Description | Data deposited in or computed by PubChem | |
Molecular Weight |
303.2 g/mol | |
Source | PubChem | |
URL | https://pubchem.ncbi.nlm.nih.gov | |
Description | Data deposited in or computed by PubChem | |
Synthesis routes and methods
Procedure details
Retrosynthesis Analysis
AI-Powered Synthesis Planning: Our tool employs the Template_relevance Pistachio, Template_relevance Bkms_metabolic, Template_relevance Pistachio_ringbreaker, Template_relevance Reaxys, Template_relevance Reaxys_biocatalysis model, leveraging a vast database of chemical reactions to predict feasible synthetic routes.
One-Step Synthesis Focus: Specifically designed for one-step synthesis, it provides concise and direct routes for your target compounds, streamlining the synthesis process.
Accurate Predictions: Utilizing the extensive PISTACHIO, BKMS_METABOLIC, PISTACHIO_RINGBREAKER, REAXYS, REAXYS_BIOCATALYSIS database, our tool offers high-accuracy predictions, reflecting the latest in chemical research and data.
Strategy Settings
Precursor scoring | Relevance Heuristic |
---|---|
Min. plausibility | 0.01 |
Model | Template_relevance |
Template Set | Pistachio/Bkms_metabolic/Pistachio_ringbreaker/Reaxys/Reaxys_biocatalysis |
Top-N result to add to graph | 6 |
Feasible Synthetic Routes
Disclaimer and Information on In-Vitro Research Products
Please be aware that all articles and product information presented on BenchChem are intended solely for informational purposes. The products available for purchase on BenchChem are specifically designed for in-vitro studies, which are conducted outside of living organisms. In-vitro studies, derived from the Latin term "in glass," involve experiments performed in controlled laboratory settings using cells or tissues. It is important to note that these products are not categorized as medicines or drugs, and they have not received approval from the FDA for the prevention, treatment, or cure of any medical condition, ailment, or disease. We must emphasize that any form of bodily introduction of these products into humans or animals is strictly prohibited by law. It is essential to adhere to these guidelines to ensure compliance with legal and ethical standards in research and experimentation.