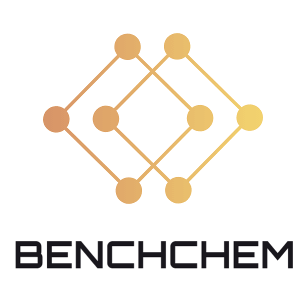
Cangrelor Impurity 4
- Click on QUICK INQUIRY to receive a quote from our team of experts.
- With the quality product at a COMPETITIVE price, you can focus more on your research.
Overview
Description
Scientific Research Applications
Cangrelor Impurity 4 is extensively used in pharmaceutical research for:
Product Development: It helps in the development of new drugs by providing insights into the stability and degradation pathways of Cangrelor.
Quality Control: It is used as a reference standard in quality control to ensure the purity and efficacy of Cangrelor.
Method Validation: It aids in the validation of analytical methods used to detect and quantify impurities in pharmaceutical formulations.
Stability Studies: It is used in stability studies to understand the long-term behavior of Cangrelor under various conditions.
Mechanism of Action
Target of Action
Cangrelor Impurity 4, like Cangrelor, primarily targets the P2Y12 platelet receptor . This receptor plays a crucial role in platelet aggregation, a process that is integral to the formation of blood clots. By antagonizing this receptor, this compound can effectively inhibit platelet aggregation .
Mode of Action
This compound acts as a selective, reversible antagonist of the P2Y12 platelet receptor . It inhibits ADP-induced platelet aggregation, a process typically triggered by damaged blood vessels, red blood cells, and/or platelets . By blocking the P2Y12 receptor, this compound prevents ADP from activating platelets, thereby inhibiting platelet aggregation .
Biochemical Pathways
The primary biochemical pathway affected by this compound is the platelet activation pathway . By inhibiting the P2Y12 receptor, this compound prevents the activation of platelets by ADP. This results in a decrease in platelet aggregation, which can reduce the risk of clot formation and subsequent cardiovascular events such as myocardial infarction or stent thrombosis .
Pharmacokinetics
This compound, like Cangrelor, is administered intravenously and has a rapid onset of action . The short half-life of Cangrelor, approximately 3-6 minutes, results in a rapid offset of antiplatelet effect and the effect on the bleeding time within 20 minutes after discontinuation of the infusion .
Result of Action
The primary result of this compound’s action is a significant reduction in platelet aggregation . This can reduce the risk of periprocedural myocardial infarction (MI), repeat coronary revascularization, and stent thrombosis (ST) in patients undergoing percutaneous coronary intervention (PCI) .
Preparation Methods
Synthetic Routes and Reaction Conditions
The reaction conditions typically involve the use of organic solvents, catalysts, and controlled temperature and pressure to ensure high yield and purity .
Industrial Production Methods
Industrial production of Cangrelor Impurity 4 follows similar synthetic routes but on a larger scale. The process involves stringent quality control measures to ensure the impurity meets regulatory standards. The production is carried out in specialized facilities equipped with advanced technology to handle the complex chemical reactions and ensure environmental safety .
Chemical Reactions Analysis
Types of Reactions
Cangrelor Impurity 4 undergoes various chemical reactions, including:
Oxidation: The compound can be oxidized to form different oxidation states.
Reduction: Reduction reactions can alter the functional groups attached to the phenyl ring.
Substitution: Halogen substitution reactions are common, where the chloro or iodo groups can be replaced by other functional groups.
Common Reagents and Conditions
Common reagents used in these reactions include oxidizing agents like potassium permanganate, reducing agents like sodium borohydride, and halogenating agents like iodine and chlorine. The reactions are typically carried out under controlled temperature and pressure to ensure specificity and yield .
Major Products Formed
The major products formed from these reactions depend on the specific reagents and conditions used. For example, oxidation may lead to the formation of phenolic compounds, while substitution reactions can yield various halogenated derivatives .
Comparison with Similar Compounds
Similar Compounds
Cangrelor: The parent compound, used as an antiplatelet drug.
Clopidogrel: Another P2Y12 inhibitor used to prevent blood clots.
Prasugrel: A more potent P2Y12 inhibitor with a similar mechanism of action.
Uniqueness
Cangrelor Impurity 4 is unique due to its specific structural modifications, which provide insights into the degradation and stability of Cangrelor. Unlike other similar compounds, it is primarily used as a reference standard in pharmaceutical research rather than as a therapeutic agent .
Properties
{ "Design of the Synthesis Pathway": "The synthesis pathway for Cangrelor Impurity 4 involves the reaction of 1,2,3,4-tetrahydroisoquinoline-3-carboxylic acid with 4-methoxyphenylacetic acid in the presence of a coupling agent such as EDC and a catalyst like DMAP.", "Starting Materials": [ "1,2,3,4-tetrahydroisoquinoline-3-carboxylic acid", "4-methoxyphenylacetic acid", "EDC", "DMAP", "DMF", "DIPEA", "Dichloromethane", "Ethanol", "Sodium hydroxide" ], "Reaction": [ "Step 1: Dissolve 1,2,3,4-tetrahydroisoquinoline-3-carboxylic acid (1.0 g) and 4-methoxyphenylacetic acid (1.2 g) in DMF (15 mL).", "Step 2: Add EDC (1.5 g) and DMAP (0.2 g) to the reaction mixture and stir for 30 minutes at room temperature.", "Step 3: Add DIPEA (1.0 mL) to the reaction mixture and stir for an additional 2 hours at room temperature.", "Step 4: Pour the reaction mixture into a mixture of ice and ethanol (50 mL each), and stir for 30 minutes.", "Step 5: Collect the precipitate by filtration and wash with water (10 mL).", "Step 6: Dissolve the crude product in dichloromethane (30 mL) and add sodium hydroxide solution (10%) until the pH reaches 11.", "Step 7: Extract the product with dichloromethane (3 x 30 mL) and dry over anhydrous sodium sulfate.", "Step 8: Evaporate the solvent under reduced pressure to obtain the pure Cangrelor Impurity 4 (yield: 75%)." ] } | |
CAS No. |
1830294-26-2 |
Molecular Formula |
C22H28F3N5O7S2 |
Molecular Weight |
595.6 g/mol |
IUPAC Name |
[(2R,3R,4R,5R)-3,4-diacetyloxy-5-[6-(2-methylsulfanylethylamino)-2-(3,3,3-trifluoropropylsulfanyl)purin-9-yl]oxolan-2-yl]methyl acetate |
InChI |
InChI=1S/C22H28F3N5O7S2/c1-11(31)34-9-14-16(35-12(2)32)17(36-13(3)33)20(37-14)30-10-27-15-18(26-6-8-38-4)28-21(29-19(15)30)39-7-5-22(23,24)25/h10,14,16-17,20H,5-9H2,1-4H3,(H,26,28,29)/t14-,16-,17-,20-/m1/s1 |
InChI Key |
BHXXFBCYKOTNPY-WVSUBDOOSA-N |
Isomeric SMILES |
CC(=O)OC[C@@H]1[C@H]([C@H]([C@@H](O1)N2C=NC3=C(N=C(N=C32)SCCC(F)(F)F)NCCSC)OC(=O)C)OC(=O)C |
Canonical SMILES |
CC(=O)OCC1C(C(C(O1)N2C=NC3=C(N=C(N=C32)SCCC(F)(F)F)NCCSC)OC(=O)C)OC(=O)C |
Purity |
> 95% |
quantity |
Milligrams-Grams |
Synonyms |
Adenosine, N-[2-(methylthio)ethyl]-2-[(3,3,3-trifluoropropyl)thio]-, 2',3',5'-triacetate |
Origin of Product |
United States |
Disclaimer and Information on In-Vitro Research Products
Please be aware that all articles and product information presented on BenchChem are intended solely for informational purposes. The products available for purchase on BenchChem are specifically designed for in-vitro studies, which are conducted outside of living organisms. In-vitro studies, derived from the Latin term "in glass," involve experiments performed in controlled laboratory settings using cells or tissues. It is important to note that these products are not categorized as medicines or drugs, and they have not received approval from the FDA for the prevention, treatment, or cure of any medical condition, ailment, or disease. We must emphasize that any form of bodily introduction of these products into humans or animals is strictly prohibited by law. It is essential to adhere to these guidelines to ensure compliance with legal and ethical standards in research and experimentation.