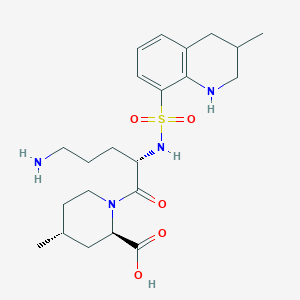
Argatroban Impurity B
Overview
Description
Argatroban Impurity B is a related substance of Argatroban, a synthetic, reversible, and competitive thrombin inhibitor. Argatroban is used as an anticoagulant for the prevention and treatment of thrombosis in patients with heparin-induced thrombocytopenia. This compound is one of the degradation products formed during the synthesis and storage of Argatroban .
Mechanism of Action
Target of Action
Argatroban, the parent compound of Argatroban Impurity B, is a synthetic, direct, selective thrombin inhibitor . Thrombin, a serine protease, plays a central role in the coagulation cascade, and its inhibition prevents the formation of blood clots .
Mode of Action
Argatroban exerts its anticoagulant effects by inhibiting thrombin-catalyzed or -induced reactions, including fibrin formation; activation of coagulation factors V, VIII, and XIII; protein C; and platelet aggregation . It is capable of inhibiting the action of both free and clot-associated thrombin .
Biochemical Pathways
The inhibition of thrombin by Argatroban impacts several biochemical pathways. It prevents the conversion of fibrinogen to fibrin, thereby inhibiting the formation of blood clots. It also inhibits the activation of coagulation factors V, VIII, and XIII, and protein C, all of which are involved in the coagulation cascade .
Pharmacokinetics
It is administered parenterally, and its anticoagulant effects are produced as plasma concentrations begin to rise . Steady-state levels of both drug and anticoagulant effect are typically attained within 1 to 3 hours and are maintained until the infusion is discontinued or the dosage adjusted .
Result of Action
The inhibition of thrombin by Argatroban results in anticoagulant, anti-inflammatory, and potentially antiviral activities . It prevents the formation of blood clots, reduces inflammation, and may have potential therapeutic benefits in viral infections .
Action Environment
The action of Argatroban can be influenced by various environmental factors. For instance, the presence of other medications can impact its efficacy. Furthermore, patient-specific factors such as the presence of sepsis can influence the dosing and effectiveness of Argatroban .
Biochemical Analysis
Biochemical Properties
Argatroban Impurity B, like Argatroban, may interact with various enzymes and proteins in the body. Argatroban is known to inhibit thrombin, a key enzyme in the coagulation cascade . It is possible that this compound may have similar interactions, although specific studies on this impurity are limited.
Cellular Effects
Argatroban has been shown to have significant effects on cells, particularly in the context of thrombosis . It can influence cell function by impacting cell signaling pathways, gene expression, and cellular metabolism .
Molecular Mechanism
Argatroban exerts its effects at the molecular level by inhibiting thrombin-catalyzed or -induced reactions, including fibrin formation and activation of coagulation factors . It is possible that this compound may have similar mechanisms of action.
Temporal Effects in Laboratory Settings
Studies on Argatroban have shown that it has a rapid onset of action and its effects can be observed within minutes of administration .
Dosage Effects in Animal Models
Studies on Argatroban have shown that it can reduce stroke damage in animal models .
Metabolic Pathways
Argatroban is metabolized in the liver via hydroxylation and aromatization of the 3-methyltetrahydroquinoline ring .
Transport and Distribution
Argatroban is known to be distributed throughout the body and can cross the placenta .
Subcellular Localization
Studies on similar compounds suggest that they may localize to various cellular compartments depending on their specific roles and interactions .
Preparation Methods
The synthesis of Argatroban Impurity B involves the hydrolysis of Argatroban under specific conditions. One method includes adding Argatroban to a reaction vessel with hydrochloric acid and water, followed by the addition of immobilized arginase and manganese chloride tetrahydrate. The reaction is carried out at a controlled temperature and stirring speed until the desired impurity is formed . Industrial production methods often involve high-performance liquid chromatography (HPLC) to separate and quantify the impurities .
Chemical Reactions Analysis
Argatroban Impurity B undergoes various chemical reactions, including hydrolysis and oxidation. Common reagents used in these reactions include hydrochloric acid, arginase, and manganese chloride. The major products formed from these reactions are the hydrolyzed and oxidized forms of Argatroban . The reactions are typically carried out under controlled conditions to ensure the formation of the desired impurity.
Scientific Research Applications
Argatroban Impurity B is primarily used in scientific research to study the stability and degradation pathways of Argatroban. It is also used in the development and validation of analytical methods for the quantification of impurities in pharmaceutical formulations. Additionally, it serves as a reference standard in quality control laboratories to ensure the purity and efficacy of Argatroban products .
Properties
IUPAC Name |
(2R,4R)-1-[(2S)-5-amino-2-[(3-methyl-1,2,3,4-tetrahydroquinolin-8-yl)sulfonylamino]pentanoyl]-4-methylpiperidine-2-carboxylic acid | |
---|---|---|
Source | PubChem | |
URL | https://pubchem.ncbi.nlm.nih.gov | |
Description | Data deposited in or computed by PubChem | |
InChI |
InChI=1S/C22H34N4O5S/c1-14-8-10-26(18(12-14)22(28)29)21(27)17(6-4-9-23)25-32(30,31)19-7-3-5-16-11-15(2)13-24-20(16)19/h3,5,7,14-15,17-18,24-25H,4,6,8-13,23H2,1-2H3,(H,28,29)/t14-,15?,17+,18-/m1/s1 | |
Source | PubChem | |
URL | https://pubchem.ncbi.nlm.nih.gov | |
Description | Data deposited in or computed by PubChem | |
InChI Key |
ZNDKSTYNIAVIGX-IOVMHBDKSA-N | |
Source | PubChem | |
URL | https://pubchem.ncbi.nlm.nih.gov | |
Description | Data deposited in or computed by PubChem | |
Canonical SMILES |
CC1CCN(C(C1)C(=O)O)C(=O)C(CCCN)NS(=O)(=O)C2=CC=CC3=C2NCC(C3)C | |
Source | PubChem | |
URL | https://pubchem.ncbi.nlm.nih.gov | |
Description | Data deposited in or computed by PubChem | |
Isomeric SMILES |
C[C@@H]1CCN([C@H](C1)C(=O)O)C(=O)[C@H](CCCN)NS(=O)(=O)C2=CC=CC3=C2NCC(C3)C | |
Source | PubChem | |
URL | https://pubchem.ncbi.nlm.nih.gov | |
Description | Data deposited in or computed by PubChem | |
Molecular Formula |
C22H34N4O5S | |
Source | PubChem | |
URL | https://pubchem.ncbi.nlm.nih.gov | |
Description | Data deposited in or computed by PubChem | |
Molecular Weight |
466.6 g/mol | |
Source | PubChem | |
URL | https://pubchem.ncbi.nlm.nih.gov | |
Description | Data deposited in or computed by PubChem | |
Retrosynthesis Analysis
AI-Powered Synthesis Planning: Our tool employs the Template_relevance Pistachio, Template_relevance Bkms_metabolic, Template_relevance Pistachio_ringbreaker, Template_relevance Reaxys, Template_relevance Reaxys_biocatalysis model, leveraging a vast database of chemical reactions to predict feasible synthetic routes.
One-Step Synthesis Focus: Specifically designed for one-step synthesis, it provides concise and direct routes for your target compounds, streamlining the synthesis process.
Accurate Predictions: Utilizing the extensive PISTACHIO, BKMS_METABOLIC, PISTACHIO_RINGBREAKER, REAXYS, REAXYS_BIOCATALYSIS database, our tool offers high-accuracy predictions, reflecting the latest in chemical research and data.
Strategy Settings
Precursor scoring | Relevance Heuristic |
---|---|
Min. plausibility | 0.01 |
Model | Template_relevance |
Template Set | Pistachio/Bkms_metabolic/Pistachio_ringbreaker/Reaxys/Reaxys_biocatalysis |
Top-N result to add to graph | 6 |
Feasible Synthetic Routes
Disclaimer and Information on In-Vitro Research Products
Please be aware that all articles and product information presented on BenchChem are intended solely for informational purposes. The products available for purchase on BenchChem are specifically designed for in-vitro studies, which are conducted outside of living organisms. In-vitro studies, derived from the Latin term "in glass," involve experiments performed in controlled laboratory settings using cells or tissues. It is important to note that these products are not categorized as medicines or drugs, and they have not received approval from the FDA for the prevention, treatment, or cure of any medical condition, ailment, or disease. We must emphasize that any form of bodily introduction of these products into humans or animals is strictly prohibited by law. It is essential to adhere to these guidelines to ensure compliance with legal and ethical standards in research and experimentation.