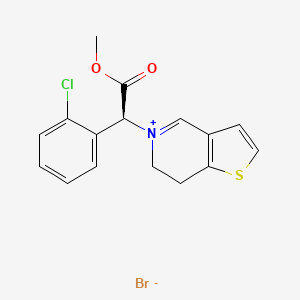
Clopidogrel Impurity 2 (Clopidogrel Iminium Impurity)
Overview
Description
Synthesis Analysis
The synthesis of Clopidogrel Impurity 2 involves starting with the raw material o-chlorobenzene glycine. Esterification obtains racemic O-chlorobenzene glycine methyl ester, which is then split with tartrate to obtain R- (-)-O-chlorobenzene glycine methyl ester. The reaction of thiophene ethanol p-toluenesulfonic esters is followed by hydrochloric acid salification to obtain ®-2- (2 thiophene ethyl amine base) (2-chloro-phenyl-) methyl acetate hydrochloride. Finally, formaldehyde cyclisation is used to obtain the localized impurity of clopidogrel liquid phase .Molecular Structure Analysis
The molecular structure of Clopidogrel Impurity 2 is represented by the formula C15H14ClNO2S . The InChI representation is InChI=1S/C16H16ClNO2S/c1-20-16(19)15(12-4-2-3-5-13(12)17)18-8-6-14-11(10-18)7-9-21-14/h2-5,7,9,15H,6,8,10H2,1H3/t15-/m0/s1 .Scientific Research Applications
Selective Oxidation and NMR Study
A study by Krake and Baumann (2021) explored the selective transformation of clopidogrel hydrogen sulfate by reactive halogen species. This process led to the formation of various degradation products, including a halogenated endo-iminium product, which is a known form of clopidogrel iminium impurity. Nuclear magnetic resonance spectroscopy was used to monitor the reaction and characterize the products (Krake & Baumann, 2021).
Characterization of Oxidation Impurity
Mohan et al. (2008) identified and characterized a principal oxidation impurity of clopidogrel. This impurity was isolated using preparative HPLC and characterized through various spectral data, contributing to the understanding of clopidogrel impurity profiles (Mohan et al., 2008).
Capillary Electrophoresis for Separation
Fayed et al. (2009) employed capillary zone electrophoresis to separate clopidogrel bisulfate from its impurities, including impurity 2. This method optimized factors like buffer concentration and pH for effective separation, providing a technique for analyzing clopidogrel impurities (Fayed et al., 2009).
TLC Method for Impurity Determination
Antić et al. (2007) developed a simple TLC method for separating clopidogrel and its hydrolytic product, SR 26334, a major impurity. This method facilitates the quantitative analysis and purity control of clopidogrel in various forms (Antić et al., 2007).
Stability-Indicating UFLC Method
Nagavi and Gurupadayya (2016) developed a stability-indicating ultra-fast LC method for separating and estimating impurities in clopidogrel. This method was validated according to ICH guidelines and is useful for quality control in pharmaceutical formulations (Nagavi & Gurupadayya, 2016).
Comparison of Original and Copy Tablets
Gomez et al. (2004) compared original PLAVIX tablets with 18 copies for impurity profile and other characteristics. This study used enantiospecific liquid chromatography to determine impurities, contributing to quality control in pharmaceutical products (Gomez et al., 2004).
Mechanism of Action
The mechanism of action of Clopidogrel, the parent compound of Clopidogrel Impurity 2, involves the irreversible inhibition of the P2Y12 ADP receptor on platelets. This prevents ADP from binding to the P2Y12 receptors, thereby inhibiting the activation of the glycoprotein GPIIb/IIIa complex and platelet aggregation .
properties
IUPAC Name |
methyl (2S)-2-(2-chlorophenyl)-2-(6,7-dihydrothieno[3,2-c]pyridin-5-ium-5-yl)acetate;bromide | |
---|---|---|
Source | PubChem | |
URL | https://pubchem.ncbi.nlm.nih.gov | |
Description | Data deposited in or computed by PubChem | |
InChI |
InChI=1S/C16H15ClNO2S.BrH/c1-20-16(19)15(12-4-2-3-5-13(12)17)18-8-6-14-11(10-18)7-9-21-14;/h2-5,7,9-10,15H,6,8H2,1H3;1H/q+1;/p-1/t15-;/m0./s1 | |
Source | PubChem | |
URL | https://pubchem.ncbi.nlm.nih.gov | |
Description | Data deposited in or computed by PubChem | |
InChI Key |
KEWLMQVQNGJIKW-RSAXXLAASA-M | |
Source | PubChem | |
URL | https://pubchem.ncbi.nlm.nih.gov | |
Description | Data deposited in or computed by PubChem | |
Canonical SMILES |
COC(=O)C(C1=CC=CC=C1Cl)[N+]2=CC3=C(CC2)SC=C3.[Br-] | |
Source | PubChem | |
URL | https://pubchem.ncbi.nlm.nih.gov | |
Description | Data deposited in or computed by PubChem | |
Isomeric SMILES |
COC(=O)[C@H](C1=CC=CC=C1Cl)[N+]2=CC3=C(CC2)SC=C3.[Br-] | |
Source | PubChem | |
URL | https://pubchem.ncbi.nlm.nih.gov | |
Description | Data deposited in or computed by PubChem | |
Molecular Formula |
C16H15BrClNO2S | |
Source | PubChem | |
URL | https://pubchem.ncbi.nlm.nih.gov | |
Description | Data deposited in or computed by PubChem | |
Molecular Weight |
400.7 g/mol | |
Source | PubChem | |
URL | https://pubchem.ncbi.nlm.nih.gov | |
Description | Data deposited in or computed by PubChem | |
CAS RN |
1396607-49-0 | |
Record name | Clopidogrel pyridinium bromide | |
Source | ChemIDplus | |
URL | https://pubchem.ncbi.nlm.nih.gov/substance/?source=chemidplus&sourceid=1396607490 | |
Description | ChemIDplus is a free, web search system that provides access to the structure and nomenclature authority files used for the identification of chemical substances cited in National Library of Medicine (NLM) databases, including the TOXNET system. | |
Record name | CLOPIDOGREL PYRIDINIUM BROMIDE | |
Source | FDA Global Substance Registration System (GSRS) | |
URL | https://gsrs.ncats.nih.gov/ginas/app/beta/substances/NNE7AU6LVL | |
Description | The FDA Global Substance Registration System (GSRS) enables the efficient and accurate exchange of information on what substances are in regulated products. Instead of relying on names, which vary across regulatory domains, countries, and regions, the GSRS knowledge base makes it possible for substances to be defined by standardized, scientific descriptions. | |
Explanation | Unless otherwise noted, the contents of the FDA website (www.fda.gov), both text and graphics, are not copyrighted. They are in the public domain and may be republished, reprinted and otherwise used freely by anyone without the need to obtain permission from FDA. Credit to the U.S. Food and Drug Administration as the source is appreciated but not required. | |
Retrosynthesis Analysis
AI-Powered Synthesis Planning: Our tool employs the Template_relevance Pistachio, Template_relevance Bkms_metabolic, Template_relevance Pistachio_ringbreaker, Template_relevance Reaxys, Template_relevance Reaxys_biocatalysis model, leveraging a vast database of chemical reactions to predict feasible synthetic routes.
One-Step Synthesis Focus: Specifically designed for one-step synthesis, it provides concise and direct routes for your target compounds, streamlining the synthesis process.
Accurate Predictions: Utilizing the extensive PISTACHIO, BKMS_METABOLIC, PISTACHIO_RINGBREAKER, REAXYS, REAXYS_BIOCATALYSIS database, our tool offers high-accuracy predictions, reflecting the latest in chemical research and data.
Strategy Settings
Precursor scoring | Relevance Heuristic |
---|---|
Min. plausibility | 0.01 |
Model | Template_relevance |
Template Set | Pistachio/Bkms_metabolic/Pistachio_ringbreaker/Reaxys/Reaxys_biocatalysis |
Top-N result to add to graph | 6 |
Feasible Synthetic Routes
Disclaimer and Information on In-Vitro Research Products
Please be aware that all articles and product information presented on BenchChem are intended solely for informational purposes. The products available for purchase on BenchChem are specifically designed for in-vitro studies, which are conducted outside of living organisms. In-vitro studies, derived from the Latin term "in glass," involve experiments performed in controlled laboratory settings using cells or tissues. It is important to note that these products are not categorized as medicines or drugs, and they have not received approval from the FDA for the prevention, treatment, or cure of any medical condition, ailment, or disease. We must emphasize that any form of bodily introduction of these products into humans or animals is strictly prohibited by law. It is essential to adhere to these guidelines to ensure compliance with legal and ethical standards in research and experimentation.