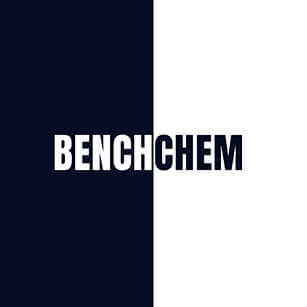
Gefitinib 3,4-Difluoro Impurity HCl
- Click on QUICK INQUIRY to receive a quote from our team of experts.
- With the quality product at a COMPETITIVE price, you can focus more on your research.
Overview
Description
Gefitinib 3,4-Difluoro Impurity Hydrochloride is a chemical compound used primarily as a reference standard in pharmaceutical research. It is a derivative of gefitinib, a tyrosine kinase inhibitor used in the treatment of non-small cell lung cancer. The chemical name for this compound is N-(3,4-Difluorophenyl)-7-methoxy-6-(3-morpholinopropoxy)quinazolin-4-amine hydrochloride .
Preparation Methods
Synthetic Routes and Reaction Conditions: The synthesis of Gefitinib 3,4-Difluoro Impurity Hydrochloride involves multiple steps, starting with the preparation of the quinazoline core structure. The key steps include:
Formation of the quinazoline core: This is typically achieved through the cyclization of appropriate precursors under controlled conditions.
Introduction of the 3,4-difluorophenyl group: This step involves the substitution reaction where the difluorophenyl group is introduced to the quinazoline core.
Attachment of the morpholinopropoxy group: This is done through a nucleophilic substitution reaction, where the morpholinopropoxy group is attached to the quinazoline core.
Methoxylation: The methoxy group is introduced through a methylation reaction.
Industrial Production Methods: Industrial production of Gefitinib 3,4-Difluoro Impurity Hydrochloride follows similar synthetic routes but on a larger scale. The process involves:
Optimization of reaction conditions: Ensuring high yield and purity.
Use of industrial-grade reagents: To maintain consistency and cost-effectiveness.
Purification: Using techniques such as crystallization, chromatography, and recrystallization to achieve the desired purity
Types of Reactions:
Oxidation: Gefitinib 3,4-Difluoro Impurity Hydrochloride can undergo oxidation reactions, leading to the formation of various oxidized products.
Reduction: The compound can be reduced under specific conditions to yield reduced derivatives.
Substitution: It can undergo nucleophilic and electrophilic substitution reactions, where different functional groups can be introduced or replaced.
Common Reagents and Conditions:
Oxidizing agents: Such as hydrogen peroxide, potassium permanganate.
Reducing agents: Such as sodium borohydride, lithium aluminum hydride.
Substitution reagents: Such as halogens, alkylating agents.
Major Products:
Oxidized derivatives: Formed through oxidation reactions.
Reduced derivatives: Formed through reduction reactions.
Substituted derivatives: Formed through substitution reactions
Scientific Research Applications
Gefitinib 3,4-Difluoro Impurity Hydrochloride is used extensively in scientific research, including:
Pharmaceutical research: As a reference standard for the analysis of gefitinib and its impurities.
Analytical chemistry: For calibration and validation of analytical methods such as high-performance liquid chromatography (HPLC).
Biological studies: To study the metabolism and degradation pathways of gefitinib.
Medicinal chemistry: For the development of new derivatives and analogs with improved therapeutic properties
Mechanism of Action
Gefitinib 3,4-Difluoro Impurity Hydrochloride, being a derivative of gefitinib, shares a similar mechanism of action. It inhibits the epidermal growth factor receptor (EGFR) tyrosine kinase by binding to the adenosine triphosphate (ATP)-binding site of the enzyme. This inhibition prevents the phosphorylation of tyrosine residues on the EGFR, thereby blocking the downstream signaling pathways that lead to cell proliferation and survival. This mechanism is particularly effective in cancer cells that overexpress EGFR .
Comparison with Similar Compounds
Gefitinib 3,4-Difluoro Impurity Hydrochloride can be compared with other similar compounds such as:
Gefitinib: The parent compound, used as a tyrosine kinase inhibitor in cancer treatment.
Erlotinib: Another tyrosine kinase inhibitor with a similar mechanism of action.
Lapatinib: A dual tyrosine kinase inhibitor targeting both EGFR and HER2.
Uniqueness: Gefitinib 3,4-Difluoro Impurity Hydrochloride is unique due to the presence of the 3,4-difluorophenyl group, which distinguishes it from other impurities and analogs. This structural difference can influence its reactivity and interaction with biological targets .
Properties
CAS No. |
184475-68-1 |
---|---|
Molecular Formula |
C22H25F2N4O3Cl |
Molecular Weight |
466.92 |
Purity |
> 95% |
quantity |
Milligrams-Grams |
Origin of Product |
United States |
Disclaimer and Information on In-Vitro Research Products
Please be aware that all articles and product information presented on BenchChem are intended solely for informational purposes. The products available for purchase on BenchChem are specifically designed for in-vitro studies, which are conducted outside of living organisms. In-vitro studies, derived from the Latin term "in glass," involve experiments performed in controlled laboratory settings using cells or tissues. It is important to note that these products are not categorized as medicines or drugs, and they have not received approval from the FDA for the prevention, treatment, or cure of any medical condition, ailment, or disease. We must emphasize that any form of bodily introduction of these products into humans or animals is strictly prohibited by law. It is essential to adhere to these guidelines to ensure compliance with legal and ethical standards in research and experimentation.