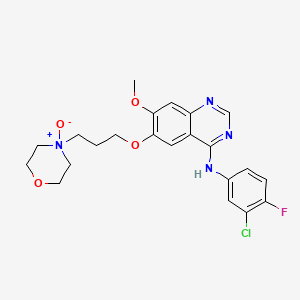
Gefitinib N-Oxide
- Click on QUICK INQUIRY to receive a quote from our team of experts.
- With the quality product at a COMPETITIVE price, you can focus more on your research.
Overview
Description
Gefitinib N-Oxide is a derivative of gefitinib, a well-known tyrosine kinase inhibitor used primarily in the treatment of non-small cell lung cancer. This compound retains the core structure of gefitinib but includes an additional oxygen atom, forming an N-oxide group. This modification can potentially alter its pharmacokinetic and pharmacodynamic properties, making it a subject of interest in medicinal chemistry and drug development.
Mechanism of Action
Target of Action
Gefitinib N-Oxide, similar to Gefitinib, primarily targets the Epidermal Growth Factor Receptor (EGFR) . EGFR is a member of a family of receptors (ErbB) which includes Her1 (EGFR), Her2 (erb-B2), Her3 (erb-B3), and Her4 (Erb-B4) . EGFR is often overexpressed in certain types of human carcinoma cells, such as lung and breast cancer cells .
Mode of Action
This compound acts as an inhibitor of the EGFR tyrosine kinase . It binds to the adenosine triphosphate (ATP)-binding site of the enzyme . By inhibiting EGFR tyrosine kinase, the downstream signaling cascades are also inhibited, resulting in inhibited malignant cell proliferation .
Biochemical Pathways
This compound affects several biochemical pathways. It perturbs signal transduction pathways, apoptosis, cell cycle, FOXO-mediated transcription, p53 signaling pathway, and metabolic pathways . Notably, it has been observed that gefitinib can cause opposite expression patterns of genes associated with metabolism of lipids and cholesterol biosynthesis in different organoids .
Pharmacokinetics
This compound, like Gefitinib, is extensively metabolized in the liver, primarily by CYP3A4, but CYP3A5 and CYP2D6 also play minor roles in its metabolism . Excretion is predominantly via the feces, with renal elimination accounting for less than 4% of the administered dose . The systemic exposure to gefitinib in elderly patients was slightly higher than that in younger patients .
Result of Action
The result of this compound’s action is the inhibition of malignant cell proliferation . By selectively targeting the mutant proteins in malignant cells, it interrupts signaling through the EGFR in target cells . Therefore, it is only effective in cancers with mutated and overactive EGFR .
Biochemical Analysis
Biochemical Properties
Gefitinib N-Oxide, like its parent compound Gefitinib, is expected to interact with EGFR, a protein that plays a crucial role in cell signaling pathways involved in cell proliferation and survival . By inhibiting EGFR, this compound could potentially interfere with these signaling pathways, thereby affecting the growth and survival of cancer cells .
Cellular Effects
This compound is likely to have similar cellular effects as Gefitinib. Gefitinib has been shown to inhibit the intracellular phosphorylation of numerous tyrosine kinases associated with transmembrane cell surface receptors, including the tyrosine kinases associated with EGFR . This inhibition can affect various cellular processes, including cell signaling pathways, gene expression, and cellular metabolism .
Molecular Mechanism
The molecular mechanism of action of this compound is expected to be similar to that of Gefitinib. Gefitinib is an inhibitor of the EGFR tyrosine kinase that binds to the adenosine triphosphate (ATP)-binding site of the enzyme . This binding inhibits the activation of EGFR, thereby disrupting the downstream signaling pathways that promote cell proliferation and survival .
Temporal Effects in Laboratory Settings
Studies on Gefitinib have shown that it can have long-term effects on cellular function . For instance, Gefitinib treatment has been shown to cause NSCLC cells to undergo apoptosis following activation of the caspase 8 cascade .
Dosage Effects in Animal Models
Studies on Gefitinib have shown that its effects can vary with dosage . For instance, weekly dosing with Gefitinib had similar or better efficacy than daily dosing in pre-clinical models of NSCLC .
Metabolic Pathways
This compound is likely to be involved in similar metabolic pathways as Gefitinib. Gefitinib is primarily metabolized in the liver via CYP3A4 . The major metabolic changes include metabolism of the N-propoxymorpholino-group, demethylation of the methoxy-substituent on the quinazoline, and oxidative defluorination of the halogenated phenyl group .
Transport and Distribution
Gefitinib has been shown to distribute extensively in most tissues, except for the brain .
Subcellular Localization
Studies on Gefitinib have shown that it can cause the translocation of certain proteins from the nucleus to the cytoplasm . For instance, Gefitinib treatment has been shown to cause the translocation of the cyclin-dependent kinase inhibitor p27 from the nucleus to the cytoplasm .
Preparation Methods
Synthetic Routes and Reaction Conditions: The synthesis of gefitinib N-Oxide typically involves the oxidation of gefitinib. One common method is the use of oxidizing agents such as hydrogen peroxide or m-chloroperbenzoic acid. The reaction is usually carried out in an organic solvent like dichloromethane at room temperature. The process can be summarized as follows:
- Dissolve gefitinib in dichloromethane.
- Add the oxidizing agent (e.g., hydrogen peroxide or m-chloroperbenzoic acid).
- Stir the reaction mixture at room temperature until the reaction is complete.
- Isolate the product by filtration or extraction, followed by purification using techniques like column chromatography.
Industrial Production Methods: Industrial production of this compound would likely follow a similar synthetic route but on a larger scale. The process would involve optimization of reaction conditions to maximize yield and purity, as well as the implementation of efficient purification and isolation techniques to ensure the final product meets pharmaceutical standards.
Chemical Reactions Analysis
Types of Reactions: Gefitinib N-Oxide can undergo various chemical reactions, including:
Reduction: The N-oxide group can be reduced back to the parent amine using reducing agents like zinc and acetic acid.
Substitution: The presence of the N-oxide group can influence electrophilic and nucleophilic substitution reactions on the aromatic ring.
Oxidation: Further oxidation can lead to the formation of more oxidized species, although this is less common.
Common Reagents and Conditions:
Reduction: Zinc and acetic acid, or other mild reducing agents.
Substitution: Electrophilic or nucleophilic reagents under appropriate conditions (e.g., halogenation using chlorine or bromine in the presence of a catalyst).
Oxidation: Strong oxidizing agents like peracids.
Major Products:
Reduction: Gefitinib (parent compound).
Substitution: Various substituted derivatives depending on the reagents used.
Oxidation: Higher oxidation states of the compound, though these are less common.
Scientific Research Applications
Gefitinib N-Oxide has several applications in scientific research:
Chemistry: Used as a model compound to study the effects of N-oxide groups on the chemical reactivity and stability of pharmaceuticals.
Biology: Investigated for its potential biological activity and interactions with cellular targets.
Medicine: Explored as a potential therapeutic agent with modified pharmacokinetic properties compared to gefitinib.
Industry: Used in the development of new drug formulations and as a reference standard in analytical chemistry.
Comparison with Similar Compounds
Gefitinib N-Oxide can be compared with other similar compounds, such as:
Gefitinib: The parent compound, a well-established EGFR inhibitor.
Erlotinib: Another EGFR inhibitor with a similar mechanism of action.
Afatinib: An irreversible EGFR inhibitor with a broader spectrum of activity.
Uniqueness: this compound’s uniqueness lies in its N-oxide group, which can alter its chemical and biological properties. This modification can potentially lead to differences in pharmacokinetics, pharmacodynamics, and overall therapeutic efficacy compared to its parent compound and other similar drugs.
By understanding the detailed chemistry and applications of this compound, researchers can explore its potential as a novel therapeutic agent and its role in advancing medicinal chemistry.
Properties
IUPAC Name |
N-(3-chloro-4-fluorophenyl)-7-methoxy-6-[3-(4-oxidomorpholin-4-ium-4-yl)propoxy]quinazolin-4-amine |
Source
|
---|---|---|
Source | PubChem | |
URL | https://pubchem.ncbi.nlm.nih.gov | |
Description | Data deposited in or computed by PubChem | |
InChI |
InChI=1S/C22H24ClFN4O4/c1-30-20-13-19-16(12-21(20)32-8-2-5-28(29)6-9-31-10-7-28)22(26-14-25-19)27-15-3-4-18(24)17(23)11-15/h3-4,11-14H,2,5-10H2,1H3,(H,25,26,27) |
Source
|
Source | PubChem | |
URL | https://pubchem.ncbi.nlm.nih.gov | |
Description | Data deposited in or computed by PubChem | |
InChI Key |
FIKOGZWCACSBHA-UHFFFAOYSA-N |
Source
|
Source | PubChem | |
URL | https://pubchem.ncbi.nlm.nih.gov | |
Description | Data deposited in or computed by PubChem | |
Canonical SMILES |
COC1=C(C=C2C(=C1)N=CN=C2NC3=CC(=C(C=C3)F)Cl)OCCC[N+]4(CCOCC4)[O-] |
Source
|
Source | PubChem | |
URL | https://pubchem.ncbi.nlm.nih.gov | |
Description | Data deposited in or computed by PubChem | |
Molecular Formula |
C22H24ClFN4O4 |
Source
|
Source | PubChem | |
URL | https://pubchem.ncbi.nlm.nih.gov | |
Description | Data deposited in or computed by PubChem | |
Molecular Weight |
462.9 g/mol |
Source
|
Source | PubChem | |
URL | https://pubchem.ncbi.nlm.nih.gov | |
Description | Data deposited in or computed by PubChem | |
Disclaimer and Information on In-Vitro Research Products
Please be aware that all articles and product information presented on BenchChem are intended solely for informational purposes. The products available for purchase on BenchChem are specifically designed for in-vitro studies, which are conducted outside of living organisms. In-vitro studies, derived from the Latin term "in glass," involve experiments performed in controlled laboratory settings using cells or tissues. It is important to note that these products are not categorized as medicines or drugs, and they have not received approval from the FDA for the prevention, treatment, or cure of any medical condition, ailment, or disease. We must emphasize that any form of bodily introduction of these products into humans or animals is strictly prohibited by law. It is essential to adhere to these guidelines to ensure compliance with legal and ethical standards in research and experimentation.