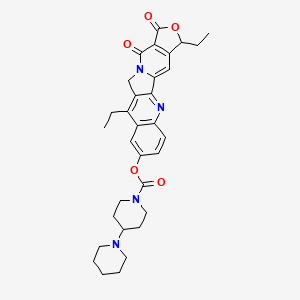
Irinotecan Lactone Impurity
Overview
Description
Irinotecan Lactone Impurity: is a byproduct associated with the synthesis and degradation of irinotecan, a chemotherapeutic agent used primarily in the treatment of colorectal cancer. Irinotecan is a prodrug that is converted into its active metabolite, SN-38, which inhibits the enzyme topoisomerase I, leading to DNA damage and cell death in cancer cells . The lactone form of irinotecan is the active form, while the carboxylate form is inactive .
Mechanism of Action
Target of Action
The primary target of Irinotecan, from which the Irinotecan Lactone Impurity is derived, is topoisomerase I . This enzyme plays a crucial role in DNA replication by relaxing torsionally strained (supercoiled) DNA .
Mode of Action
Upon administration, Irinotecan is converted into its active metabolite, SN-38 , by carboxylesterases in the liver and gastrointestinal tract . Both Irinotecan and SN-38 inhibit DNA topoisomerase I, acting on the S and G2 phases of the cell cycle . They bind to the topoisomerase I-DNA complex and prevent the religation of the DNA strands, thereby interfering with DNA synthesis . This leads to the arrest of the cell cycle in the S-G2 phase and cancer cell death .
Pharmacokinetics
Irinotecan therapy is characterized by several dose-limiting toxicities and large interindividual pharmacokinetic variability . The clearance of Irinotecan is mainly biliary (66%) and independent of dose, estimated at 12–21 L/h/m2 . Conversion of Irinotecan lactone to carboxylate within the circulation is rapid, with an initial half-life of between 9 and 14 min, which results in a 50% reduction in Irinotecan lactone concentration after 2.5 h, compared with end of infusion .
Result of Action
The result of Irinotecan’s action is the arrest of the cell cycle in the S-G2 phase, leading to cancer cell death . The lactone forms of Irinotecan and SN-38 were transported mainly by a passive diffusion, whereas the carboxylate forms were absorbed as a result of an active transport mechanism .
Action Environment
Irinotecan metabolism is prone to environmental and genetic influences . Genetic variants in the DNA of these enzymes and transporters could predict a part of the drug-related toxicity and efficacy of treatment . Patient characteristics, lifestyle, and comedication also influence Irinotecan pharmacokinetics . Other factors, including dietary restriction, are currently being studied .
Biochemical Analysis
Biochemical Properties
Irinotecan Lactone Impurity plays a crucial role in biochemical reactions. It is involved in the complex metabolism of irinotecan, a topoisomerase I inhibitor . The metabolism of irinotecan involves hydrolyzation by carboxylesterases to its active metabolite SN-38, which is 100- to 1000-fold more active compared with irinotecan itself .
Cellular Effects
The effects of this compound on cells are primarily related to its role in the metabolism of irinotecan. The active metabolite SN-38, produced from irinotecan, has a significant impact on cell function, including effects on cell signaling pathways, gene expression, and cellular metabolism .
Molecular Mechanism
The molecular mechanism of action of this compound is closely tied to its role in the metabolism of irinotecan. The conversion of irinotecan to its active metabolite SN-38 involves binding interactions with carboxylesterases, leading to enzyme activation and changes in gene expression .
Temporal Effects in Laboratory Settings
The effects of this compound over time in laboratory settings are complex and can involve changes in the product’s stability and degradation . Long-term effects on cellular function have been observed in in vitro or in vivo studies .
Dosage Effects in Animal Models
The effects of this compound can vary with different dosages in animal models . High doses of irinotecan, from which this compound is derived, can lead to toxic or adverse effects .
Metabolic Pathways
This compound is involved in the metabolic pathways of irinotecan. This includes interactions with enzymes such as carboxylesterases, cytochrome P450 (CYP) 3A4, and uridine diphosphate glucuronosyltransferase (UGT) 1A .
Transport and Distribution
The transport and distribution of this compound within cells and tissues are influenced by various factors. These include interactions with transporters and binding proteins, as well as effects on its localization or accumulation .
Subcellular Localization
Current knowledge suggests that it may be involved in specific targeting signals or post-translational modifications that direct it to specific compartments or organelles .
Preparation Methods
Synthetic Routes and Reaction Conditions: The synthesis of irinotecan involves the reaction of SN-38 with bipiperidyl-carbonyl chloride or phosgene to form irinotecan . The reaction conditions typically involve the use of organic solvents and controlled temperatures to ensure the formation of the desired product. The lactone impurity can form during these reactions due to incomplete conversion or side reactions.
Industrial Production Methods: Industrial production of irinotecan involves large-scale synthesis using similar reaction conditions as in laboratory settings but optimized for higher yields and purity. The process includes rigorous purification steps to remove impurities, including the lactone impurity .
Chemical Reactions Analysis
Types of Reactions: Irinotecan lactone impurity undergoes several types of chemical reactions, including:
Hydrolysis: The lactone ring can hydrolyze to form the carboxylate form, especially under basic conditions.
Oxidation and Reduction: These reactions can occur under specific conditions, altering the chemical structure and activity of the compound.
Substitution: The lactone ring can participate in substitution reactions, leading to the formation of various derivatives.
Common Reagents and Conditions:
Hydrolysis: Typically involves water or aqueous solutions under acidic or basic conditions.
Oxidation: Common oxidizing agents include hydrogen peroxide and potassium permanganate.
Reduction: Reducing agents such as sodium borohydride can be used.
Substitution: Various nucleophiles can be used depending on the desired substitution product.
Major Products:
Hydrolysis: Formation of the carboxylate form.
Oxidation and Reduction: Formation of oxidized or reduced derivatives.
Substitution: Formation of substituted lactone derivatives.
Scientific Research Applications
Chemistry: Irinotecan lactone impurity is studied to understand the stability and degradation pathways of irinotecan. This knowledge helps in improving the synthesis and storage conditions of the drug .
Biology and Medicine: Research on this compound helps in understanding the pharmacokinetics and pharmacodynamics of irinotecan. It also aids in identifying potential side effects and toxicities associated with the impurity .
Industry: In the pharmaceutical industry, controlling the levels of this compound is crucial for ensuring the safety and efficacy of irinotecan formulations .
Comparison with Similar Compounds
SN-38: The active metabolite of irinotecan with higher potency.
Topotecan: Another topoisomerase I inhibitor used in cancer treatment.
Camptothecin: The parent compound from which irinotecan is derived.
Uniqueness: Irinotecan lactone impurity is unique in its formation and presence as a byproduct in irinotecan formulations. Its presence can affect the overall efficacy and safety of the drug, making its control and study important in pharmaceutical development .
Biological Activity
Irinotecan, a chemotherapeutic agent, is primarily used in the treatment of various cancers, including colorectal cancer. Its efficacy is largely attributed to its active metabolite, SN-38, which exists in two forms: a lactone form that is pharmacologically active and a carboxylate form that is inactive. The conversion between these forms is critical for the drug's therapeutic effectiveness. This article focuses on the biological activity of irinotecan lactone impurity, examining its pharmacokinetics, mechanisms of action, and clinical implications.
This compound has a molecular formula of and is structurally related to irinotecan. The lactone form is favored at lower pH levels, while the carboxylate form predominates at higher pH levels. This pH-dependent equilibrium plays a significant role in the drug's stability and activity in biological systems .
Irinotecan acts primarily as an inhibitor of topoisomerase I, an enzyme crucial for DNA replication. The active lactone form stabilizes the DNA-topoisomerase I complex, leading to DNA damage and subsequent cell death. In contrast, the carboxylate form lacks this inhibitory activity .
Pharmacokinetics
The pharmacokinetics of irinotecan and its metabolites are complex and influenced by various factors:
- Conversion Rates : The conversion of irinotecan lactone to carboxylate occurs rapidly in vivo, with a half-life of approximately 9.5 minutes. This rapid conversion can affect therapeutic outcomes if not managed properly .
- Distribution : Irinotecan has a volume of distribution ranging from 110 to 234 L/m², with significant plasma protein binding (30-68% for irinotecan and up to 95% for SN-38) . The binding affinity for plasma proteins varies between the two forms, with the lactone form exhibiting higher binding capacity.
- Elimination : The drug is primarily eliminated via biliary excretion, with renal excretion accounting for a smaller proportion. Approximately 25% of irinotecan is excreted unchanged in bile within 48 hours .
Stability and Delivery Methods
Research indicates that the stability of irinotecan's active lactone form can be enhanced through specific delivery methods:
- Drug Eluting Beads : Studies have shown that irinotecan can be effectively delivered using drug-eluting beads that stabilize the lactone form through charge interactions with sulfonate groups in the hydrogel matrix. This method prolongs the half-life of the active form and reduces toxicity associated with systemic administration .
- pH Influence : The stability of the lactone form is significantly affected by pH levels; maintaining an acidic environment can favor its retention and enhance therapeutic efficacy .
Case Studies
Several clinical studies have examined the impacts of this compound on treatment outcomes:
- Colorectal Cancer Treatment : A study involving patients treated with irinotecan showed that those with higher plasma concentrations of SN-38 (primarily in its lactone form) experienced better treatment responses compared to those with lower concentrations .
- Pharmacogenomic Variability : Variations in patient metabolism due to genetic differences in enzyme activity (e.g., UGT1A1 polymorphisms) have been linked to differential responses to irinotecan therapy, emphasizing the importance of understanding individual pharmacokinetics when administering this drug .
Properties
IUPAC Name |
(5,13-diethyl-7,9-dioxo-6-oxa-10,20-diazapentacyclo[10.8.0.02,10.04,8.014,19]icosa-1(20),2,4(8),12,14(19),15,17-heptaen-16-yl) 4-piperidin-1-ylpiperidine-1-carboxylate | |
---|---|---|
Source | PubChem | |
URL | https://pubchem.ncbi.nlm.nih.gov | |
Description | Data deposited in or computed by PubChem | |
InChI |
InChI=1S/C32H36N4O5/c1-3-21-22-16-20(40-32(39)35-14-10-19(11-15-35)34-12-6-5-7-13-34)8-9-25(22)33-29-24(21)18-36-26(29)17-23-27(4-2)41-31(38)28(23)30(36)37/h8-9,16-17,19,27H,3-7,10-15,18H2,1-2H3 | |
Source | PubChem | |
URL | https://pubchem.ncbi.nlm.nih.gov | |
Description | Data deposited in or computed by PubChem | |
InChI Key |
MPKLBQKGQFVIOG-UHFFFAOYSA-N | |
Source | PubChem | |
URL | https://pubchem.ncbi.nlm.nih.gov | |
Description | Data deposited in or computed by PubChem | |
Canonical SMILES |
CCC1C2=C(C(=O)N3CC4=C(C5=C(C=CC(=C5)OC(=O)N6CCC(CC6)N7CCCCC7)N=C4C3=C2)CC)C(=O)O1 | |
Source | PubChem | |
URL | https://pubchem.ncbi.nlm.nih.gov | |
Description | Data deposited in or computed by PubChem | |
Molecular Formula |
C32H36N4O5 | |
Source | PubChem | |
URL | https://pubchem.ncbi.nlm.nih.gov | |
Description | Data deposited in or computed by PubChem | |
DSSTOX Substance ID |
DTXSID20729786 | |
Record name | 3,10-Diethyl-1,13-dioxo-11,13-dihydro-1H,3H-furo[3',4':6,7]indolizino[1,2-b]quinolin-8-yl [1,4'-bipiperidine]-1'-carboxylate | |
Source | EPA DSSTox | |
URL | https://comptox.epa.gov/dashboard/DTXSID20729786 | |
Description | DSSTox provides a high quality public chemistry resource for supporting improved predictive toxicology. | |
Molecular Weight |
556.7 g/mol | |
Source | PubChem | |
URL | https://pubchem.ncbi.nlm.nih.gov | |
Description | Data deposited in or computed by PubChem | |
CAS No. |
143490-53-3 | |
Record name | 3,10-Diethyl-1,13-dioxo-11,13-dihydro-1H,3H-furo[3',4':6,7]indolizino[1,2-b]quinolin-8-yl [1,4'-bipiperidine]-1'-carboxylate | |
Source | EPA DSSTox | |
URL | https://comptox.epa.gov/dashboard/DTXSID20729786 | |
Description | DSSTox provides a high quality public chemistry resource for supporting improved predictive toxicology. | |
Disclaimer and Information on In-Vitro Research Products
Please be aware that all articles and product information presented on BenchChem are intended solely for informational purposes. The products available for purchase on BenchChem are specifically designed for in-vitro studies, which are conducted outside of living organisms. In-vitro studies, derived from the Latin term "in glass," involve experiments performed in controlled laboratory settings using cells or tissues. It is important to note that these products are not categorized as medicines or drugs, and they have not received approval from the FDA for the prevention, treatment, or cure of any medical condition, ailment, or disease. We must emphasize that any form of bodily introduction of these products into humans or animals is strictly prohibited by law. It is essential to adhere to these guidelines to ensure compliance with legal and ethical standards in research and experimentation.