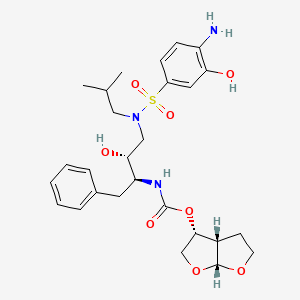
3'-Hydroxy Darunavir
Overview
Description
3’-Hydroxy Darunavir is a derivative of Darunavir, an antiretroviral medication used primarily in the treatment and prevention of human immunodeficiency virus (HIV) infection. Darunavir belongs to the protease inhibitor class of drugs, which work by inhibiting the action of the HIV protease enzyme, thereby preventing viral replication. The addition of a hydroxyl group at the 3’ position enhances the compound’s pharmacological properties, potentially improving its efficacy and stability.
Mechanism of Action
Target of Action
3’-Hydroxy Darunavir, also known as Darunavir, is primarily targeted towards the HIV-1 protease , an enzyme crucial for the life-cycle of the HIV-1 virus . This protease is responsible for cleaving the polyprotein precursors, which leads to the formation of mature infectious HIV particles. By inhibiting this protease, Darunavir prevents the maturation of HIV particles, thereby controlling the spread of the virus .
Mode of Action
Darunavir is a nonpeptidic inhibitor of the HIV-1 protease . It binds to the active site of the protease through a number of hydrogen bonds, thereby inhibiting its function . As a second-generation protease inhibitor, Darunavir is designed to combat resistance to standard HIV therapy .
Biochemical Pathways
The primary biochemical pathway affected by Darunavir is the HIV-1 viral replication cycle . By inhibiting the HIV-1 protease, Darunavir prevents the cleavage of polyprotein precursors, which are necessary for the formation of mature infectious HIV particles . This results in the production of immature, non-infectious viral particles, thereby reducing the viral load and controlling the spread of the virus within the body .
Pharmacokinetics
Darunavir is primarily metabolized by cytochrome P450 3A (CYP3A) isoenzymes . It is often co-administered with low-dose ritonavir, which is an inhibitor of CYP3A isoenzymes. Ritonavir pharmacologically enhances Darunavir, resulting in increased plasma concentrations and allowing for a lower daily dose . The terminal elimination half-life of Darunavir is approximately 15 hours in the presence of ritonavir . The bioavailability of Darunavir increases to 82% when co-administered with ritonavir, compared with 37% when Darunavir is administered alone .
Result of Action
The primary result of Darunavir’s action is the reduction of the viral load in HIV-1 infected patients. By inhibiting the HIV-1 protease and preventing the maturation of HIV particles, Darunavir reduces the number of infectious particles in the body . This helps to control the spread of the virus and can lead to improved immune function in HIV-1 infected patients .
Action Environment
The action of Darunavir can be influenced by various environmental factors. For instance, certain substances can affect the metabolism of Darunavir. St. John’s wort, for example, may reduce the effectiveness of Darunavir by increasing the breakdown of Darunavir by the metabolic enzyme CYP3A . Therefore, the patient’s diet, lifestyle, and co-administration of other medications can all influence the action, efficacy, and stability of Darunavir .
Biochemical Analysis
Biochemical Properties
3’-Hydroxy Darunavir plays a significant role in biochemical reactions, particularly in the inhibition of the HIV protease enzyme . This enzyme is crucial for the life cycle of the HIV virus, and its inhibition prevents the virus from replicating effectively . The interaction between 3’-Hydroxy Darunavir and the HIV protease enzyme is primarily facilitated by hydrogen bonds .
Cellular Effects
3’-Hydroxy Darunavir has profound effects on various types of cells, especially those infected with HIV. It significantly decreases the viral load and increases CD4 cell counts, thereby reducing the morbidity and mortality of HIV infection . Furthermore, it has been suggested that 3’-Hydroxy Darunavir may have anti-apoptotic properties, potentially protecting cells from programmed cell death .
Molecular Mechanism
The molecular mechanism of 3’-Hydroxy Darunavir involves binding to the active site of the HIV-1 protease, thereby blocking its function . This binding is facilitated by the flexibility of the 3’-Hydroxy Darunavir molecule, allowing it to adapt to changes in the shape of the protease enzyme . This strong interaction results in the inhibition of the enzyme and the prevention of HIV replication .
Temporal Effects in Laboratory Settings
In laboratory settings, the effects of 3’-Hydroxy Darunavir have been observed over time. Studies have shown that 3’-Hydroxy Darunavir maintains its stability and effectiveness over extended periods
Dosage Effects in Animal Models
The effects of 3’-Hydroxy Darunavir in animal models vary with different dosages. While specific studies on 3’-Hydroxy Darunavir are limited, research on Darunavir has shown that it maintains its effectiveness at varying dosages, with only a small percentage of patients experiencing concentrations below the IC50 for resistant strains .
Metabolic Pathways
3’-Hydroxy Darunavir is primarily metabolized by cytochrome P450 3A (CYP3A) isoenzymes . This metabolic pathway involves oxidative metabolism, leading to the production of several metabolites . The interaction of 3’-Hydroxy Darunavir with these enzymes plays a crucial role in its pharmacokinetics and pharmacodynamics.
Transport and Distribution
3’-Hydroxy Darunavir is transported and distributed within cells and tissues. It is believed to involve an active transport process, possibly involving P-glycoprotein . The absorption of 3’-Hydroxy Darunavir increases when co-administered with low-dose ritonavir, enhancing its bioavailability .
Subcellular Localization
It is reasonable to speculate that it may follow a similar pattern to Darunavir, which is localized within the endoplasmic reticulum and within spherical, vesicular structures in the cytoplasm . These structures represent a previously undescribed subcellular compartment likely capable of synthesizing mevalonate .
Preparation Methods
Synthetic Routes and Reaction Conditions
The synthesis of 3’-Hydroxy Darunavir involves several key steps, starting from commercially available starting materials. The synthetic route typically includes the following steps:
Formation of the Core Structure: The core structure of Darunavir is synthesized through a series of reactions, including amide bond formation, sulfonamide formation, and carbamate formation.
Introduction of the Hydroxyl Group: The hydroxyl group is introduced at the 3’ position through selective hydroxylation reactions. This can be achieved using reagents such as osmium tetroxide or other hydroxylating agents under controlled conditions.
Purification and Characterization: The final product is purified using techniques such as high-performance liquid chromatography (HPLC) and characterized using spectroscopic methods like nuclear magnetic resonance (NMR) and mass spectrometry (MS).
Industrial Production Methods
Industrial production of 3’-Hydroxy Darunavir follows similar synthetic routes but on a larger scale. The process involves optimization of reaction conditions to ensure high yield and purity. Key steps include:
Batch or Continuous Flow Synthesis: Depending on the scale, batch or continuous flow synthesis methods are employed to maximize efficiency.
Catalysis and Optimization: Catalysts and reaction conditions are optimized to enhance the selectivity and yield of the desired product.
Quality Control: Rigorous quality control measures are implemented to ensure the consistency and purity of the final product.
Chemical Reactions Analysis
Types of Reactions
3’-Hydroxy Darunavir undergoes various chemical reactions, including:
Oxidation: The hydroxyl group can be oxidized to form ketones or aldehydes using oxidizing agents such as potassium permanganate or chromium trioxide.
Reduction: The compound can undergo reduction reactions to form alcohols or amines using reducing agents like lithium aluminum hydride or sodium borohydride.
Substitution: The hydroxyl group can be substituted with other functional groups through nucleophilic substitution reactions.
Common Reagents and Conditions
Oxidation: Potassium permanganate, chromium trioxide, and other oxidizing agents under acidic or basic conditions.
Reduction: Lithium aluminum hydride, sodium borohydride, and other reducing agents under anhydrous conditions.
Substitution: Nucleophiles such as halides, amines, or thiols under appropriate conditions.
Major Products Formed
Oxidation: Formation of ketones or aldehydes.
Reduction: Formation of alcohols or amines.
Substitution: Formation of substituted derivatives with various functional groups.
Scientific Research Applications
3’-Hydroxy Darunavir has several scientific research applications, including:
Chemistry: Used as a model compound for studying hydroxylation reactions and the effects of hydroxyl groups on pharmacological properties.
Biology: Investigated for its potential to inhibit HIV protease and other viral enzymes, contributing to the development of new antiviral therapies.
Medicine: Explored for its enhanced efficacy and stability compared to Darunavir, potentially leading to improved treatment options for HIV patients.
Industry: Utilized in the development of new pharmaceutical formulations and drug delivery systems.
Comparison with Similar Compounds
Similar Compounds
Darunavir: The parent compound, used widely in HIV treatment.
Fosamprenavir: Another protease inhibitor with a similar mechanism of action.
Atazanavir: A protease inhibitor with a different chemical structure but similar therapeutic use.
Uniqueness
3’-Hydroxy Darunavir is unique due to the presence of the hydroxyl group at the 3’ position, which enhances its pharmacological properties. This modification improves its binding affinity to the HIV protease enzyme, potentially leading to better efficacy and stability compared to other protease inhibitors.
Properties
IUPAC Name |
[(3aS,4R,6aR)-2,3,3a,4,5,6a-hexahydrofuro[2,3-b]furan-4-yl] N-[(2S,3R)-4-[(4-amino-3-hydroxyphenyl)sulfonyl-(2-methylpropyl)amino]-3-hydroxy-1-phenylbutan-2-yl]carbamate | |
---|---|---|
Source | PubChem | |
URL | https://pubchem.ncbi.nlm.nih.gov | |
Description | Data deposited in or computed by PubChem | |
InChI |
InChI=1S/C27H37N3O8S/c1-17(2)14-30(39(34,35)19-8-9-21(28)23(31)13-19)15-24(32)22(12-18-6-4-3-5-7-18)29-27(33)38-25-16-37-26-20(25)10-11-36-26/h3-9,13,17,20,22,24-26,31-32H,10-12,14-16,28H2,1-2H3,(H,29,33)/t20-,22-,24+,25-,26+/m0/s1 | |
Source | PubChem | |
URL | https://pubchem.ncbi.nlm.nih.gov | |
Description | Data deposited in or computed by PubChem | |
InChI Key |
JHSMVOSDDWDUEW-QSDJZFCLSA-N | |
Source | PubChem | |
URL | https://pubchem.ncbi.nlm.nih.gov | |
Description | Data deposited in or computed by PubChem | |
Canonical SMILES |
CC(C)CN(CC(C(CC1=CC=CC=C1)NC(=O)OC2COC3C2CCO3)O)S(=O)(=O)C4=CC(=C(C=C4)N)O | |
Source | PubChem | |
URL | https://pubchem.ncbi.nlm.nih.gov | |
Description | Data deposited in or computed by PubChem | |
Isomeric SMILES |
CC(C)CN(C[C@H]([C@H](CC1=CC=CC=C1)NC(=O)O[C@H]2CO[C@@H]3[C@H]2CCO3)O)S(=O)(=O)C4=CC(=C(C=C4)N)O | |
Source | PubChem | |
URL | https://pubchem.ncbi.nlm.nih.gov | |
Description | Data deposited in or computed by PubChem | |
Molecular Formula |
C27H37N3O8S | |
Source | PubChem | |
URL | https://pubchem.ncbi.nlm.nih.gov | |
Description | Data deposited in or computed by PubChem | |
Molecular Weight |
563.7 g/mol | |
Source | PubChem | |
URL | https://pubchem.ncbi.nlm.nih.gov | |
Description | Data deposited in or computed by PubChem | |
CAS No. |
1159613-24-7 | |
Record name | R-330326 | |
Source | ChemIDplus | |
URL | https://pubchem.ncbi.nlm.nih.gov/substance/?source=chemidplus&sourceid=1159613247 | |
Description | ChemIDplus is a free, web search system that provides access to the structure and nomenclature authority files used for the identification of chemical substances cited in National Library of Medicine (NLM) databases, including the TOXNET system. | |
Record name | R-330326 | |
Source | FDA Global Substance Registration System (GSRS) | |
URL | https://gsrs.ncats.nih.gov/ginas/app/beta/substances/PBF42439XY | |
Description | The FDA Global Substance Registration System (GSRS) enables the efficient and accurate exchange of information on what substances are in regulated products. Instead of relying on names, which vary across regulatory domains, countries, and regions, the GSRS knowledge base makes it possible for substances to be defined by standardized, scientific descriptions. | |
Explanation | Unless otherwise noted, the contents of the FDA website (www.fda.gov), both text and graphics, are not copyrighted. They are in the public domain and may be republished, reprinted and otherwise used freely by anyone without the need to obtain permission from FDA. Credit to the U.S. Food and Drug Administration as the source is appreciated but not required. | |
Retrosynthesis Analysis
AI-Powered Synthesis Planning: Our tool employs the Template_relevance Pistachio, Template_relevance Bkms_metabolic, Template_relevance Pistachio_ringbreaker, Template_relevance Reaxys, Template_relevance Reaxys_biocatalysis model, leveraging a vast database of chemical reactions to predict feasible synthetic routes.
One-Step Synthesis Focus: Specifically designed for one-step synthesis, it provides concise and direct routes for your target compounds, streamlining the synthesis process.
Accurate Predictions: Utilizing the extensive PISTACHIO, BKMS_METABOLIC, PISTACHIO_RINGBREAKER, REAXYS, REAXYS_BIOCATALYSIS database, our tool offers high-accuracy predictions, reflecting the latest in chemical research and data.
Strategy Settings
Precursor scoring | Relevance Heuristic |
---|---|
Min. plausibility | 0.01 |
Model | Template_relevance |
Template Set | Pistachio/Bkms_metabolic/Pistachio_ringbreaker/Reaxys/Reaxys_biocatalysis |
Top-N result to add to graph | 6 |
Feasible Synthetic Routes
Disclaimer and Information on In-Vitro Research Products
Please be aware that all articles and product information presented on BenchChem are intended solely for informational purposes. The products available for purchase on BenchChem are specifically designed for in-vitro studies, which are conducted outside of living organisms. In-vitro studies, derived from the Latin term "in glass," involve experiments performed in controlled laboratory settings using cells or tissues. It is important to note that these products are not categorized as medicines or drugs, and they have not received approval from the FDA for the prevention, treatment, or cure of any medical condition, ailment, or disease. We must emphasize that any form of bodily introduction of these products into humans or animals is strictly prohibited by law. It is essential to adhere to these guidelines to ensure compliance with legal and ethical standards in research and experimentation.