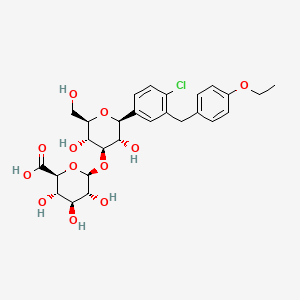
Dapagliflozin 3-o-glucuronide
- Click on QUICK INQUIRY to receive a quote from our team of experts.
- With the quality product at a COMPETITIVE price, you can focus more on your research.
Description
Dapagliflozin 3-O-β-D-glucuronide is a metabolite of dapagliflozin . Dapagliflozin is a first-generation selective sodium-glucose cotransporter (SGLT) 2 inhibitor that blocks glucose transport .
Synthesis Analysis
Dapagliflozin undergoes extensive metabolism, predominantly in the liver and kidneys by uridine diphosphate-glucuronosyltransferase-1A9 to the major metabolite dapagliflozin 3-O-glucuronide . This metabolite is not an SGLT2 inhibitor at clinically relevant exposures .Molecular Structure Analysis
The molecular formula of Dapagliflozin 3-O-glucuronide is C27H33ClO12 . More detailed information about its structure can be found in the referenced papers .Chemical Reactions Analysis
Dapagliflozin 3-O-glucuronide is primarily formed through the glucuronidation of dapagliflozin . The elimination of Dapagliflozin 3-O-glucuronide occurs mainly via renal excretion, with 61% of a dapagliflozin dose being recovered as this metabolite in urine .Scientific Research Applications
1. Treatment of Type 2 Diabetes Mellitus Dapagliflozin was originally developed as an oral glucose-lowering drug for the treatment of type 2 diabetes mellitus . It works by blocking the reabsorption of glucose in the kidneys, leading to increased urinary glucose excretion . This helps lower blood glucose levels in patients with type 2 diabetes mellitus .
Cardiovascular Benefits
Emerging data suggest that cardiovascular benefits extend to patients without diabetes . This could be a potential area of research for Dapagliflozin and its metabolites.
Chronic Kidney Disease
Dapagliflozin has been evaluated for its pharmacokinetics and exposure-response relationship in patients with chronic kidney disease without type 2 diabetes . The study found that plasma exposure increased with decreasing kidney function .
Reduction of HbA1c Levels
In randomized, double-blind, placebo-controlled trials, Dapagliflozin as add-on therapy to other medications significantly reduced HbA1c from baseline versus placebo . This shows its potential in managing blood sugar levels in diabetic patients.
Blood Pressure Regulation
A post hoc analysis of one study suggested that systolic blood pressure was lowered with Dapagliflozin to a greater degree in patients receiving a β blocker or a calcium-channel blocker as their additional antihypertensive drug . This indicates a potential application of Dapagliflozin in blood pressure regulation.
Proteinuria
Every 100-ng h/mL increment in Dapagliflozin plasma exposure was associated with a decrease in the urinary albumin:creatinine ratio . This suggests that Dapagliflozin could potentially be used in the management of proteinuria.
properties
IUPAC Name |
(2S,3S,4S,5R,6R)-6-[(2S,3S,4R,5R,6R)-2-[4-chloro-3-[(4-ethoxyphenyl)methyl]phenyl]-3,5-dihydroxy-6-(hydroxymethyl)oxan-4-yl]oxy-3,4,5-trihydroxyoxane-2-carboxylic acid |
Source
|
---|---|---|
Source | PubChem | |
URL | https://pubchem.ncbi.nlm.nih.gov | |
Description | Data deposited in or computed by PubChem | |
InChI |
InChI=1S/C27H33ClO12/c1-2-37-15-6-3-12(4-7-15)9-14-10-13(5-8-16(14)28)23-22(34)24(18(30)17(11-29)38-23)39-27-21(33)19(31)20(32)25(40-27)26(35)36/h3-8,10,17-25,27,29-34H,2,9,11H2,1H3,(H,35,36)/t17-,18-,19+,20+,21-,22+,23+,24+,25+,27-/m1/s1 |
Source
|
Source | PubChem | |
URL | https://pubchem.ncbi.nlm.nih.gov | |
Description | Data deposited in or computed by PubChem | |
InChI Key |
ZYZULHSUKTZGTR-PTNNFGGUSA-N |
Source
|
Source | PubChem | |
URL | https://pubchem.ncbi.nlm.nih.gov | |
Description | Data deposited in or computed by PubChem | |
Canonical SMILES |
CCOC1=CC=C(C=C1)CC2=C(C=CC(=C2)C3C(C(C(C(O3)CO)O)OC4C(C(C(C(O4)C(=O)O)O)O)O)O)Cl |
Source
|
Source | PubChem | |
URL | https://pubchem.ncbi.nlm.nih.gov | |
Description | Data deposited in or computed by PubChem | |
Isomeric SMILES |
CCOC1=CC=C(C=C1)CC2=C(C=CC(=C2)[C@H]3[C@@H]([C@H]([C@@H]([C@H](O3)CO)O)O[C@H]4[C@@H]([C@H]([C@@H]([C@H](O4)C(=O)O)O)O)O)O)Cl |
Source
|
Source | PubChem | |
URL | https://pubchem.ncbi.nlm.nih.gov | |
Description | Data deposited in or computed by PubChem | |
Molecular Formula |
C27H33ClO12 |
Source
|
Source | PubChem | |
URL | https://pubchem.ncbi.nlm.nih.gov | |
Description | Data deposited in or computed by PubChem | |
Molecular Weight |
585.0 g/mol |
Source
|
Source | PubChem | |
URL | https://pubchem.ncbi.nlm.nih.gov | |
Description | Data deposited in or computed by PubChem | |
Product Name |
Dapagliflozin 3-o-glucuronide | |
CAS RN |
1351438-75-9 |
Source
|
Record name | Dapagliflozin 3-O-glucuronide | |
Source | ChemIDplus | |
URL | https://pubchem.ncbi.nlm.nih.gov/substance/?source=chemidplus&sourceid=1351438759 | |
Description | ChemIDplus is a free, web search system that provides access to the structure and nomenclature authority files used for the identification of chemical substances cited in National Library of Medicine (NLM) databases, including the TOXNET system. | |
Record name | DAPAGLIFLOZIN 3-O-GLUCURONIDE | |
Source | FDA Global Substance Registration System (GSRS) | |
URL | https://gsrs.ncats.nih.gov/ginas/app/beta/substances/X42P3B148J | |
Description | The FDA Global Substance Registration System (GSRS) enables the efficient and accurate exchange of information on what substances are in regulated products. Instead of relying on names, which vary across regulatory domains, countries, and regions, the GSRS knowledge base makes it possible for substances to be defined by standardized, scientific descriptions. | |
Explanation | Unless otherwise noted, the contents of the FDA website (www.fda.gov), both text and graphics, are not copyrighted. They are in the public domain and may be republished, reprinted and otherwise used freely by anyone without the need to obtain permission from FDA. Credit to the U.S. Food and Drug Administration as the source is appreciated but not required. | |
Disclaimer and Information on In-Vitro Research Products
Please be aware that all articles and product information presented on BenchChem are intended solely for informational purposes. The products available for purchase on BenchChem are specifically designed for in-vitro studies, which are conducted outside of living organisms. In-vitro studies, derived from the Latin term "in glass," involve experiments performed in controlled laboratory settings using cells or tissues. It is important to note that these products are not categorized as medicines or drugs, and they have not received approval from the FDA for the prevention, treatment, or cure of any medical condition, ailment, or disease. We must emphasize that any form of bodily introduction of these products into humans or animals is strictly prohibited by law. It is essential to adhere to these guidelines to ensure compliance with legal and ethical standards in research and experimentation.