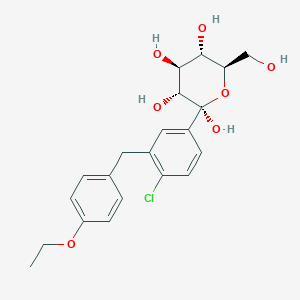
Dapagliflozin impurity
Overview
Description
Dapagliflozin impurity is a byproduct formed during the synthesis of dapagliflozin, a sodium-glucose co-transporter 2 inhibitor used in the treatment of type 2 diabetes mellitus. These impurities can arise from various stages of the synthetic process and can affect the purity and efficacy of the final pharmaceutical product .
Synthetic Routes and Reaction Conditions:
- One method involves the addition of n-butyllithium to a solution of 5-bromo-2-chloro-4’-ethyoxyl diphenylmethane to prepare a lithium reagent. This reagent then reacts with pinacol methyl borate through a Suzuki coupling reaction to form the this compound .
- Another method involves the esterification process of dapagliflozin with triethyl silanol, which generates a dapagliflozin-triethyl silyl ether impurity as a byproduct of a reduction reaction .
Industrial Production Methods:
- Industrial production methods focus on optimizing the purification process of dapagliflozin intermediates to increase reaction yield, improve product quality, and reduce raw material costs .
Types of Reactions:
Oxidation: Dapagliflozin impurities can undergo oxidation reactions, leading to the formation of various degradation products.
Reduction: Reduction reactions can also occur, particularly during the synthesis process, leading to the formation of specific impurities.
Substitution: Substitution reactions, such as the Suzuki coupling reaction, are commonly used in the synthesis of dapagliflozin impurities.
Common Reagents and Conditions:
Reagents: n-butyllithium, pinacol methyl borate, triethyl silanol.
Major Products:
Mechanism of Action
Target of Action
Dapagliflozin impurity primarily targets the sodium-glucose cotransporter 2 (SGLT2) . This protein is found in the kidneys and plays a crucial role in reabsorbing glucose from the renal filtrate back into the bloodstream .
Mode of Action
This compound acts as a highly potent, selective, and reversible inhibitor of SGLT2 . By blocking the SGLT2 protein, it reduces the reabsorption of glucose in the kidneys, leading to an increase in glucose excretion via urine . This action results in a decrease in blood glucose levels .
Biochemical Pathways
The primary biochemical pathway affected by this compound is glucose metabolism . By inhibiting SGLT2, it induces glycosuria, reduces glucose toxicity, and improves insulin sensitivity . This leads to significant changes in the downstream effects of glucose metabolism, including improved glycemic control .
Pharmacokinetics
This compound undergoes extensive metabolism and transforms into metabolites in humans . It is absorbed rapidly and reaches a maximum concentration within 2 hours . Approximately 75% of the dose is excreted in urine, and 21% is excreted in bile . The pharmacokinetics of this compound can be influenced by factors such as kidney function .
Result of Action
The primary molecular effect of this compound’s action is the reduction of blood glucose levels . On a cellular level, it leads to an increase in urinary glucose excretion . Clinically, this results in improved glycemic control in adults with type 2 diabetes mellitus .
Action Environment
The action, efficacy, and stability of this compound can be influenced by various environmental factors. For instance, it has been found to degrade significantly under acidic, alkaline, oxidative, photolytic, and dry-heat conditions . Furthermore, the Predicted Environmental Concentration (PEC) / Predicted No Effect Concentration (PNEC) ratio indicates that the use of this compound presents an insignificant risk to the environment .
Biochemical Analysis
Biochemical Properties
Dapagliflozin impurity, like its parent compound, may interact with various enzymes and proteins. It is primarily glucuronidated to an inactive 3-O-glucuronide metabolite. The nature of these interactions is likely to be similar to those of Dapagliflozin, involving binding to the SGLT2 protein .
Cellular Effects
Dapagliflozin has been shown to influence cell function by inhibiting glucose reabsorption in the proximal tubules of the kidney . It is plausible that this compound may have similar effects.
Molecular Mechanism
Dapagliflozin works by inhibiting the SGLT2 protein, reducing glucose reabsorption and leading to increased glucose excretion . This compound may have a similar mechanism of action.
Dosage Effects in Animal Models
Studies on Dapagliflozin have shown it to be effective at reducing blood glucose levels in diabetic rats .
Metabolic Pathways
This compound is involved in the glucuronidation metabolic pathway, where it is transformed into an inactive 3-O-glucuronide metabolite. This process involves the interaction with various enzymes and cofactors.
Transport and Distribution
Dapagliflozin is known to be transported to the proximal renal tubule where it exerts its effects .
Subcellular Localization
Dapagliflozin is known to localize to the proximal renal tubule, where it inhibits the SGLT2 protein .
Scientific Research Applications
Dapagliflozin impurities are studied extensively to understand their impact on the stability and efficacy of the pharmaceutical product. These studies are crucial for:
Chemistry: Understanding the chemical stability and degradation pathways of dapagliflozin.
Biology: Investigating the biological activity and potential toxicity of impurities.
Medicine: Ensuring the safety and efficacy of dapagliflozin as a therapeutic agent.
Industry: Developing improved synthesis and purification methods to minimize impurity formation
Comparison with Similar Compounds
Empagliflozin: Another sodium-glucose co-transporter 2 inhibitor used for similar therapeutic purposes.
Canagliflozin: Also a sodium-glucose co-transporter 2 inhibitor with similar applications.
Comparison:
- Dapagliflozin and empagliflozin both demonstrate significant benefits in terms of heart failure and renal outcomes. dapagliflozin has been shown to have a higher risk of hospitalization for heart failure at lower doses compared to empagliflozin .
- The impurities formed during the synthesis of these compounds can vary, affecting their stability and efficacy differently .
Biological Activity
Dapagliflozin impurity, specifically known as this compound A, is a derivative of dapagliflozin, a sodium-glucose cotransporter 2 (SGLT2) inhibitor widely used in the management of type 2 diabetes mellitus. This article explores the biological activity of this compound, including its pharmacological effects, metabolic pathways, and potential implications in clinical settings.
Overview of Dapagliflozin and Its Impurities
Dapagliflozin is primarily utilized for its ability to lower blood glucose levels by inhibiting the SGLT2 transporter in the kidneys, promoting glucose excretion through urine. However, impurities such as this compound A have raised concerns regarding their biological activity and safety profile. This impurity is classified as a genotoxic compound, which can potentially damage genetic material at low concentrations .
Biological Activity
Mechanism of Action:
- Dapagliflozin and its impurities function by inhibiting SGLT2, leading to reduced reabsorption of glucose in the renal tubules. This results in increased urinary glucose excretion and subsequently lowers blood glucose levels .
- The presence of impurities like this compound A may alter the overall pharmacodynamics and pharmacokinetics of the drug, potentially leading to unexpected biological responses.
Pharmacological Effects:
- Studies have shown that dapagliflozin effectively reduces fasting plasma glucose and improves glycemic control in diabetic patients. In animal models, it has demonstrated significant anti-hyperglycemic activity .
- Dapagliflozin's impact on metabolic pathways has been investigated through various clinical trials. For instance, a study involving heart failure patients highlighted changes in metabolite clusters associated with energy metabolism when treated with dapagliflozin .
Case Studies and Clinical Findings
1. Efficacy in Heart Failure:
A placebo-controlled trial known as DEFINE-HF assessed the effects of dapagliflozin on patients with heart failure with reduced ejection fraction (HFrEF). The study found that dapagliflozin treatment was associated with improved metabolic profiles, including increased ketone bodies and alterations in acylcarnitine levels, which are indicative of enhanced fatty acid oxidation .
2. Renal Outcomes:
The DARWIN-T2D study evaluated renal endpoints in type 2 diabetes patients treated with dapagliflozin compared to other glucose-lowering therapies. Results indicated that dapagliflozin had a favorable effect on albumin excretion rates (AER) and estimated glomerular filtration rates (eGFR), confirming its antiproteinuric effects despite a mild decline in eGFR .
Impurity Characterization and Safety Profile
Characterization:
this compound A has been characterized using advanced analytical techniques such as mass spectrometry and nuclear magnetic resonance (NMR). These methods confirm its structure and purity levels, which are critical for ensuring patient safety .
Safety Concerns:
The genotoxic nature of this compound A necessitates careful monitoring during drug development and clinical use. Regulatory agencies emphasize the importance of controlling impurities to minimize potential risks associated with genetic damage .
Summary Table: Biological Activity of this compound
Parameter | Dapagliflozin | This compound A |
---|---|---|
Mechanism of Action | SGLT2 Inhibition | Potentially altered SGLT2 effects |
Primary Use | Type 2 Diabetes | Safety concerns due to genotoxicity |
Clinical Efficacy | Reduces blood glucose | Unknown impact on efficacy |
Metabolic Effects | Improves metabolic profiles | May interfere with metabolic pathways |
Regulatory Status | Approved | Requires monitoring for safety |
Properties
IUPAC Name |
(2S,3R,4S,5S,6R)-2-[4-chloro-3-[(4-ethoxyphenyl)methyl]phenyl]-6-(hydroxymethyl)oxane-2,3,4,5-tetrol | |
---|---|---|
Source | PubChem | |
URL | https://pubchem.ncbi.nlm.nih.gov | |
Description | Data deposited in or computed by PubChem | |
InChI |
InChI=1S/C21H25ClO7/c1-2-28-15-6-3-12(4-7-15)9-13-10-14(5-8-16(13)22)21(27)20(26)19(25)18(24)17(11-23)29-21/h3-8,10,17-20,23-27H,2,9,11H2,1H3/t17-,18-,19+,20-,21+/m1/s1 | |
Source | PubChem | |
URL | https://pubchem.ncbi.nlm.nih.gov | |
Description | Data deposited in or computed by PubChem | |
InChI Key |
KYDGWGYAUCJZDV-ADAARDCZSA-N | |
Source | PubChem | |
URL | https://pubchem.ncbi.nlm.nih.gov | |
Description | Data deposited in or computed by PubChem | |
Canonical SMILES |
CCOC1=CC=C(C=C1)CC2=C(C=CC(=C2)C3(C(C(C(C(O3)CO)O)O)O)O)Cl | |
Source | PubChem | |
URL | https://pubchem.ncbi.nlm.nih.gov | |
Description | Data deposited in or computed by PubChem | |
Isomeric SMILES |
CCOC1=CC=C(C=C1)CC2=C(C=CC(=C2)[C@]3([C@@H]([C@H]([C@@H]([C@H](O3)CO)O)O)O)O)Cl | |
Source | PubChem | |
URL | https://pubchem.ncbi.nlm.nih.gov | |
Description | Data deposited in or computed by PubChem | |
Molecular Formula |
C21H25ClO7 | |
Source | PubChem | |
URL | https://pubchem.ncbi.nlm.nih.gov | |
Description | Data deposited in or computed by PubChem | |
Molecular Weight |
424.9 g/mol | |
Source | PubChem | |
URL | https://pubchem.ncbi.nlm.nih.gov | |
Description | Data deposited in or computed by PubChem | |
CAS No. |
960404-86-8 | |
Record name | 1-C-(4-Chloro-3-((4-ethoxyphenyl)methyl)phenyl)-alpha-D-glucopyranose | |
Source | ChemIDplus | |
URL | https://pubchem.ncbi.nlm.nih.gov/substance/?source=chemidplus&sourceid=0960404868 | |
Description | ChemIDplus is a free, web search system that provides access to the structure and nomenclature authority files used for the identification of chemical substances cited in National Library of Medicine (NLM) databases, including the TOXNET system. | |
Record name | 1-C-[4-Chloro-3-[(4-ethoxyphenyl)methyl]phenyl]-α-D-glucopyranose | |
Source | FDA Global Substance Registration System (GSRS) | |
URL | https://gsrs.ncats.nih.gov/ginas/app/beta/substances/2GLW9C3QQ3 | |
Description | The FDA Global Substance Registration System (GSRS) enables the efficient and accurate exchange of information on what substances are in regulated products. Instead of relying on names, which vary across regulatory domains, countries, and regions, the GSRS knowledge base makes it possible for substances to be defined by standardized, scientific descriptions. | |
Explanation | Unless otherwise noted, the contents of the FDA website (www.fda.gov), both text and graphics, are not copyrighted. They are in the public domain and may be republished, reprinted and otherwise used freely by anyone without the need to obtain permission from FDA. Credit to the U.S. Food and Drug Administration as the source is appreciated but not required. | |
Q1: What is the significance of synthesizing and studying Dapagliflozin impurities?
A1: Understanding the impurities present in a drug substance like Dapagliflozin is crucial for several reasons. [] Impurities can potentially impact the drug's safety and efficacy profiles. By synthesizing these impurities, researchers can:
Q2: Can you describe the synthetic route employed in the research paper to produce the Dapagliflozin impurity?
A2: The research paper outlines a multi-step synthesis of a this compound starting from D-glucose. [] The process involves:
Disclaimer and Information on In-Vitro Research Products
Please be aware that all articles and product information presented on BenchChem are intended solely for informational purposes. The products available for purchase on BenchChem are specifically designed for in-vitro studies, which are conducted outside of living organisms. In-vitro studies, derived from the Latin term "in glass," involve experiments performed in controlled laboratory settings using cells or tissues. It is important to note that these products are not categorized as medicines or drugs, and they have not received approval from the FDA for the prevention, treatment, or cure of any medical condition, ailment, or disease. We must emphasize that any form of bodily introduction of these products into humans or animals is strictly prohibited by law. It is essential to adhere to these guidelines to ensure compliance with legal and ethical standards in research and experimentation.