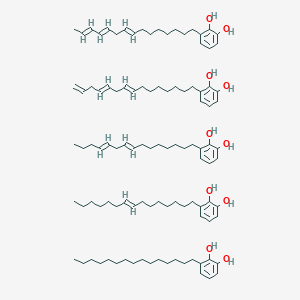
Urushiol
Overview
Description
Urushiol, also known as this compound, is a useful research compound. Its molecular formula is C21H34O2 and its molecular weight is 318.49. The purity is usually 95%.
BenchChem offers high-quality this compound suitable for many research applications. Different packaging options are available to accommodate customers' requirements. Please inquire for more information about this compound including the price, delivery time, and more detailed information at info@benchchem.com.
Properties
IUPAC Name |
3-[(8E,11E)-pentadeca-8,11-dienyl]benzene-1,2-diol;3-[(8E,11E)-pentadeca-8,11,14-trienyl]benzene-1,2-diol;3-[(8E,11E,13E)-pentadeca-8,11,13-trienyl]benzene-1,2-diol;3-[(E)-pentadec-8-enyl]benzene-1,2-diol;3-pentadecylbenzene-1,2-diol | |
---|---|---|
Source | PubChem | |
URL | https://pubchem.ncbi.nlm.nih.gov | |
Description | Data deposited in or computed by PubChem | |
InChI |
InChI=1S/C21H36O2.C21H34O2.C21H32O2.2C21H30O2/c5*1-2-3-4-5-6-7-8-9-10-11-12-13-14-16-19-17-15-18-20(22)21(19)23/h15,17-18,22-23H,2-14,16H2,1H3;7-8,15,17-18,22-23H,2-6,9-14,16H2,1H3;4-5,7-8,15,17-18,22-23H,2-3,6,9-14,16H2,1H3;2-5,7-8,15,17-18,22-23H,6,9-14,16H2,1H3;2,4-5,7-8,15,17-18,22-23H,1,3,6,9-14,16H2/b;8-7+;5-4+,8-7+;3-2+,5-4+,8-7+;5-4+,8-7+ | |
Source | PubChem | |
URL | https://pubchem.ncbi.nlm.nih.gov | |
Description | Data deposited in or computed by PubChem | |
InChI Key |
QARRXYBJLBIVAK-UEMSJJPVSA-N | |
Source | PubChem | |
URL | https://pubchem.ncbi.nlm.nih.gov | |
Description | Data deposited in or computed by PubChem | |
Canonical SMILES |
CCCCCCCCCCCCCCCC1=C(C(=CC=C1)O)O.CCCCCCC=CCCCCCCCC1=C(C(=CC=C1)O)O.CCCC=CCC=CCCCCCCCC1=C(C(=CC=C1)O)O.CC=CC=CCC=CCCCCCCCC1=C(C(=CC=C1)O)O.C=CCC=CCC=CCCCCCCCC1=C(C(=CC=C1)O)O | |
Source | PubChem | |
URL | https://pubchem.ncbi.nlm.nih.gov | |
Description | Data deposited in or computed by PubChem | |
Isomeric SMILES |
CCCCCCCCCCCCCCCC1=C(C(=CC=C1)O)O.CCCCCC/C=C/CCCCCCCC1=C(C(=CC=C1)O)O.CCC/C=C/C/C=C/CCCCCCCC1=C(C(=CC=C1)O)O.C/C=C/C=C/C/C=C/CCCCCCCC1=C(C(=CC=C1)O)O.C=CC/C=C/C/C=C/CCCCCCCC1=C(C(=CC=C1)O)O | |
Source | PubChem | |
URL | https://pubchem.ncbi.nlm.nih.gov | |
Description | Data deposited in or computed by PubChem | |
Molecular Formula |
C105H162O10 | |
Source | PubChem | |
URL | https://pubchem.ncbi.nlm.nih.gov | |
Description | Data deposited in or computed by PubChem | |
Molecular Weight |
1584.4 g/mol | |
Source | PubChem | |
URL | https://pubchem.ncbi.nlm.nih.gov | |
Description | Data deposited in or computed by PubChem | |
Physical Description |
Pale yellow liquid; [Merck Index] | |
Record name | Urushiol | |
Source | Haz-Map, Information on Hazardous Chemicals and Occupational Diseases | |
URL | https://haz-map.com/Agents/7692 | |
Description | Haz-Map® is an occupational health database designed for health and safety professionals and for consumers seeking information about the adverse effects of workplace exposures to chemical and biological agents. | |
Explanation | Copyright (c) 2022 Haz-Map(R). All rights reserved. Unless otherwise indicated, all materials from Haz-Map are copyrighted by Haz-Map(R). No part of these materials, either text or image may be used for any purpose other than for personal use. Therefore, reproduction, modification, storage in a retrieval system or retransmission, in any form or by any means, electronic, mechanical or otherwise, for reasons other than personal use, is strictly prohibited without prior written permission. | |
Boiling Point |
200 °C | |
Record name | URUSHIOL | |
Source | Hazardous Substances Data Bank (HSDB) | |
URL | https://pubchem.ncbi.nlm.nih.gov/source/hsdb/7485 | |
Description | The Hazardous Substances Data Bank (HSDB) is a toxicology database that focuses on the toxicology of potentially hazardous chemicals. It provides information on human exposure, industrial hygiene, emergency handling procedures, environmental fate, regulatory requirements, nanomaterials, and related areas. The information in HSDB has been assessed by a Scientific Review Panel. | |
Solubility |
Soluble in alcohol, ether, and benzene | |
Record name | URUSHIOL | |
Source | Hazardous Substances Data Bank (HSDB) | |
URL | https://pubchem.ncbi.nlm.nih.gov/source/hsdb/7485 | |
Description | The Hazardous Substances Data Bank (HSDB) is a toxicology database that focuses on the toxicology of potentially hazardous chemicals. It provides information on human exposure, industrial hygiene, emergency handling procedures, environmental fate, regulatory requirements, nanomaterials, and related areas. The information in HSDB has been assessed by a Scientific Review Panel. | |
Density |
0.968 | |
Record name | URUSHIOL | |
Source | Hazardous Substances Data Bank (HSDB) | |
URL | https://pubchem.ncbi.nlm.nih.gov/source/hsdb/7485 | |
Description | The Hazardous Substances Data Bank (HSDB) is a toxicology database that focuses on the toxicology of potentially hazardous chemicals. It provides information on human exposure, industrial hygiene, emergency handling procedures, environmental fate, regulatory requirements, nanomaterials, and related areas. The information in HSDB has been assessed by a Scientific Review Panel. | |
Mechanism of Action |
Allergic contact dermatitis to poison ivy (Toxicodendron radicans) is believed to be mediated by T lymphocytes specific for the hapten urushiol. Activated T lymphocytes may produce pathology by a variety of mechanisms including direct cytotoxicity, production of lymphokines, recruitment of non-specific effector cells, non-specific cytotoxicity, and possibly autologous DR reactivity. The regulation and pathogenesis of this condition was studied by cloning and characterizing urushiol-specific T cells from the peripheral blood of patients with poison ivy dermatitis. Multiple CD8+ (T8+) urushiol-specific clones were derived. All clones that proliferated in response to a crude extract of T. radicans also proliferated in response to purified urushiol. Thus, urushiol appears to be the single immunogenic component of T. radicans resin. Pentadecylcatechol (PDC), which differs from urushiol only in the lack of unsaturated bonds in its lipophilic tail, stimulated only one of seven clones tested. This suggests that the double bonds in the C15 lipophilic tail of urushiol are required for antigenicity. Several of the CD8+ urushiol-specific clone suppressed pokeweed mitogen-induced IgG production in the presence of urushiol. Suppression was triggered specifically by urushiol and required MHC compatibility both for the antigen-presenting cells and the responding B cells. These suppressor clones were isolated from convalescent blood and may represent a mechanism for the termination of an allergic contact dermatitis. [PubMed - indexed for MEDLINE], Epidermal keratinocytes in culture have been shown to produce many cytokines, and their proteins have been identified in skin tissue samples. It has therefore been assumed that these cytokines are transcribed in vivo by the epidermis in response to contact allergens. In this report, in situ hybridization was used to detect the messenger RNAs for interleukin-1 alpha (IL-1 alpha), interleukin-1 beta (IL-1 beta) and tumour necrosis factor-alpha (TNF-alpha) in samples of human skin prior to and at various times after application of urushiol, the immunogenic component of poison ivy/oak. In sensitive subjects, IL-1 alpha and TNF-alpha mRNAs showed a progressive increase in transcript levels that paralleled the clinical and histological features of the inflammatory process. The time-course of the IL-1 beta response differed from that of IL-1 alpha and TNF-alpha, in that there was an early (by 6 h after urushiol administration) elevation in IL-1 beta mRNA that occurred before there was evidence of inflammation and had returned to background levels by 72 h when the reaction had reached its peak. In contrast to urushiol-sensitive subjects, urushiol-anergic individuals did not exhibit an increase in IL-1 alpha, IL-1 beta or TNF-alpha mRNA levels. The data provide evidence for an in vivo role for epidermal IL-1 alpha, IL-1 beta and TNF-alpha transcription in the regulation of IL-1 beta and TNF-alpha polypeptide levels in the epidermis in response to this common contact allergen. [PubMed - indexed for MEDLINE], Urushiol is fat-soluble, penetrates the stratum corneum, and binds to Langerhans cells in the epidermis. These haptenated cells then migrate to lymph nodes, where T cells are activated; they then return to the skin, where they are involved in the dermatitis, The ability of small molecules such as urushiol, present as a wax on the poison ivy leaf surface, to cause allergic contact dermatitis (rhus dermatitis) has fascinated immunologists for decades. Current dogma suggests that these epicutaneously applied catechol-containing molecules serve as haptens to conjugate with larger proteins via reactive o-quinone intermediates. These complexes are then recognized as foreign antigens by the immune system and elicit a hypersensitivity reaction. Phorbol ester can directly induce cultured keratinocyte (KC) intercellular adhesion molecule-1 (ICAM-1) expression via a protein kinase C (PK-C)-dependent mechanism. As urushiol is also a known PK-C agonist, we asked if topical application of a poison ivy/oak mixture could directly induce epidermal KC ICAM-1 expression. During the pre-erythematous phase of this reaction (4 to 20 hours), epidermal KCs expressed ICAM-1; this "initiation phase" preceded the appearance of activated memory T lymphocytes in the papillary dermis, and thus appeared to be nonlymphokine mediated. A near-contiguous cellular-adhesion molecular network was identified by ICAM-1 staining of basal KCs, dermal dendrocytes, and endothelial cells. During the second 24-hour period with the onset of erythema and edema, there was an "amplification phase" of more intense KC ICAM-1 expression coupled with relatively weak KC HLA-DR expression that coincided with dermal and epidermal T-cell infiltration. This suggests the presence of lymphokines, such as gamma interferon, during the amplification phase because of KC HLA-DR expression. On cultured KCs, urushiol directly induced ICAM-1 expression but not HLA-DR. Thus, in addition to functioning as an antigenic hapten, urushiol directly induces KC ICAM-1 expression. The KC ICAM-1 expression may then alter the dynamic trafficking of memory T cells in the epidermis, so as to initiate cutaneous inflammation in a nonantigen specific manner. This initiation phase is followed by T-cell infiltration and consequent lymphokine production that significantly amplifies the original stimulus. Thus much can still be learned about the molecular pathophysiology of this common type of cutaneous inflammation. [PubMed - indexed for MEDLINE] | |
Record name | URUSHIOL | |
Source | Hazardous Substances Data Bank (HSDB) | |
URL | https://pubchem.ncbi.nlm.nih.gov/source/hsdb/7485 | |
Description | The Hazardous Substances Data Bank (HSDB) is a toxicology database that focuses on the toxicology of potentially hazardous chemicals. It provides information on human exposure, industrial hygiene, emergency handling procedures, environmental fate, regulatory requirements, nanomaterials, and related areas. The information in HSDB has been assessed by a Scientific Review Panel. | |
Color/Form |
Pale yellow liquid | |
CAS No. |
53237-59-5 | |
Record name | Urushiol | |
Source | ChemIDplus | |
URL | https://pubchem.ncbi.nlm.nih.gov/substance/?source=chemidplus&sourceid=0053237595 | |
Description | ChemIDplus is a free, web search system that provides access to the structure and nomenclature authority files used for the identification of chemical substances cited in National Library of Medicine (NLM) databases, including the TOXNET system. | |
Record name | URUSHIOL | |
Source | Hazardous Substances Data Bank (HSDB) | |
URL | https://pubchem.ncbi.nlm.nih.gov/source/hsdb/7485 | |
Description | The Hazardous Substances Data Bank (HSDB) is a toxicology database that focuses on the toxicology of potentially hazardous chemicals. It provides information on human exposure, industrial hygiene, emergency handling procedures, environmental fate, regulatory requirements, nanomaterials, and related areas. The information in HSDB has been assessed by a Scientific Review Panel. | |
Disclaimer and Information on In-Vitro Research Products
Please be aware that all articles and product information presented on BenchChem are intended solely for informational purposes. The products available for purchase on BenchChem are specifically designed for in-vitro studies, which are conducted outside of living organisms. In-vitro studies, derived from the Latin term "in glass," involve experiments performed in controlled laboratory settings using cells or tissues. It is important to note that these products are not categorized as medicines or drugs, and they have not received approval from the FDA for the prevention, treatment, or cure of any medical condition, ailment, or disease. We must emphasize that any form of bodily introduction of these products into humans or animals is strictly prohibited by law. It is essential to adhere to these guidelines to ensure compliance with legal and ethical standards in research and experimentation.