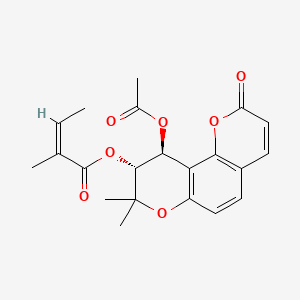
(+/-)-Praeruptorin A
- Click on QUICK INQUIRY to receive a quote from our team of experts.
- With the quality product at a COMPETITIVE price, you can focus more on your research.
Overview
Description
(+/-)-Praeruptorin A is a natural compound isolated from the roots of Peucedanum praeruptorum Dunn, a traditional Chinese medicinal herb. It belongs to the class of coumarins, which are known for their diverse biological activities. This compound has garnered significant attention due to its potential therapeutic properties, including anti-inflammatory, anti-cancer, and cardiovascular benefits.
Mechanism of Action
Praeruptorin A, also known as [(9R,10S)-10-acetyloxy-8,8-dimethyl-2-oxo-9,10-dihydropyrano[2,3-f]chromen-9-yl] (Z)-2-methylbut-2-enoate, DL-Praeruptorin A, or (-)-Praeruptorin A, is a bioactive compound isolated from the traditional Chinese medicinal plant Peucedanum praeruptorum Dunn . This compound has been reported to exhibit a wide range of pharmacological activities.
Target of Action
Praeruptorin A primarily targets the NFATc1 and NF-κB pathways . It also interacts with p38 and Akt signaling pathways . These targets play crucial roles in various biological processes, including osteoclast differentiation, inflammation, and cellular stress responses .
Mode of Action
Praeruptorin A inhibits the RANKL-stimulated osteoclast differentiation by inhibiting p38 and Akt signaling pathways . This leads to a downregulated expression of c-Fos and NFATc1, transcription factors that regulate osteoclast-specific genes . Additionally, Praeruptorin A exerts anti-inflammatory effects in vitro through inhibition of NF-κB activation .
Biochemical Pathways
Praeruptorin A affects several biochemical pathways. It inhibits the p38/Akt-c-Fos-NFATc1 signaling pathway and PLCγ-independent Ca2+ oscillation , which are involved in osteoclast differentiation . It also inhibits the activation of the NF-κB pathway , thereby reducing the expression of inflammatory factors
Biochemical Analysis
Biochemical Properties
Praeruptorin A plays a crucial role in various biochemical reactions. It interacts with several enzymes and proteins, influencing their activity and function. One of the primary interactions is with the NF-κB signaling pathway, where Praeruptorin A inhibits the activation of NF-κB, thereby exerting its anti-inflammatory effects . Additionally, Praeruptorin A has been shown to modulate the activity of matrix metalloproteinase-2 (MMP-2) and tissue inhibitor of metalloproteinase-2 (TIMP-2), which are involved in extracellular matrix remodeling . These interactions highlight the compound’s potential in regulating inflammatory responses and tissue repair processes.
Cellular Effects
Praeruptorin A exhibits significant effects on various cell types and cellular processes. In human cervical cancer cells, Praeruptorin A inhibits cell proliferation, migration, and invasion by suppressing MMP-2 expression and ERK1/2 signaling . It also induces cell cycle arrest at the G0/G1 phase and modulates the expression of cell cycle-related proteins such as Rb, p16, p21, and p27 . Furthermore, Praeruptorin A has been shown to have age-related differential effects on human fibroblasts, influencing cell growth, survival, and metabolic activity . These cellular effects underscore the compound’s potential in cancer therapy and anti-aging interventions.
Molecular Mechanism
The molecular mechanism of Praeruptorin A involves several key interactions at the molecular level. Praeruptorin A binds to and inhibits the activation of NF-κB, a transcription factor involved in the regulation of inflammatory responses . This inhibition leads to a decrease in the expression of pro-inflammatory cytokines and other inflammatory mediators. Additionally, Praeruptorin A suppresses the ERK1/2 signaling pathway, which is crucial for cell proliferation and survival . By modulating these pathways, Praeruptorin A exerts its anti-inflammatory and anti-tumor effects.
Temporal Effects in Laboratory Settings
In laboratory settings, the effects of Praeruptorin A have been observed to change over time. Studies have shown that Praeruptorin A induces vasodilation in isolated rat thoracic aorta by influencing endothelium-derived relaxing factors through the NO-cGMP and prostacyclin pathways The stability and degradation of Praeruptorin A in various experimental conditions have also been investigated, revealing its potential for long-term therapeutic applications
Dosage Effects in Animal Models
The effects of Praeruptorin A vary with different dosages in animal models. In studies involving isolated rat thoracic aorta, Praeruptorin A exhibited dose-dependent vasodilatory effects . At higher doses, Praeruptorin A significantly reduced vasoconstriction by inhibiting Ca2+ inflow . Additionally, the compound’s anti-tumor effects have been observed at specific concentrations, with higher doses leading to a more pronounced inhibition of cancer cell proliferation and invasion . These findings highlight the importance of dosage optimization for therapeutic applications.
Metabolic Pathways
Praeruptorin A is involved in several metabolic pathways, primarily mediated by cytochrome P450 enzymes. In rat liver microsomes, Praeruptorin A undergoes hydroxylation, resulting in the formation of multiple metabolites . The major CYP isoform responsible for its metabolism is CYP3A1/2, which acts in a concentration-dependent manner . Understanding these metabolic pathways is crucial for predicting drug-drug interactions and optimizing the pharmacokinetics of Praeruptorin A.
Transport and Distribution
The transport and distribution of Praeruptorin A within cells and tissues have been studied extensively. In rats, Praeruptorin A exhibits a fast distribution phase followed by a slower elimination phase . The highest concentrations of Praeruptorin A are observed in the lung, heart, liver, and kidney, with detectable levels in the brain, indicating its ability to cross the blood-brain barrier . These findings provide insights into the compound’s potential therapeutic applications and its distribution profile in vivo.
Subcellular Localization
Praeruptorin A’s subcellular localization plays a significant role in its activity and function. While specific targeting signals or post-translational modifications directing Praeruptorin A to particular compartments or organelles have not been fully elucidated, its interactions with key signaling pathways suggest its presence in the cytoplasm and nucleus
Preparation Methods
Synthetic Routes and Reaction Conditions
The synthesis of (+/-)-Praeruptorin A involves several steps, starting from simple precursors. One common synthetic route includes the condensation of umbelliferone with appropriate aldehydes under basic conditions, followed by cyclization and further functional group modifications. The reaction conditions typically involve the use of solvents like ethanol or methanol and catalysts such as piperidine or pyridine.
Industrial Production Methods
Industrial production of this compound often relies on the extraction from natural sources, particularly the roots of Peucedanum praeruptorum Dunn. The extraction process involves drying and grinding the roots, followed by solvent extraction using ethanol or methanol. The crude extract is then purified using chromatographic techniques to isolate this compound.
Chemical Reactions Analysis
Types of Reactions
(+/-)-Praeruptorin A undergoes various chemical reactions, including:
Oxidation: It can be oxidized to form hydroxylated derivatives.
Reduction: Reduction reactions can lead to the formation of dihydro derivatives.
Substitution: Substitution reactions, particularly nucleophilic substitutions, can modify the coumarin ring.
Common Reagents and Conditions
Oxidation: Reagents like potassium permanganate or hydrogen peroxide under acidic or basic conditions.
Reduction: Catalytic hydrogenation using palladium on carbon or sodium borohydride.
Substitution: Nucleophiles such as amines or thiols in the presence of a base like sodium hydroxide.
Major Products
The major products formed from these reactions include hydroxylated, dihydro, and substituted derivatives of this compound, each with potentially different biological activities.
Scientific Research Applications
(+/-)-Praeruptorin A has been extensively studied for its scientific research applications:
Chemistry: Used as a precursor for synthesizing other coumarin derivatives.
Biology: Investigated for its role in modulating biological pathways and cellular functions.
Medicine: Explored for its anti-inflammatory, anti-cancer, and cardiovascular protective effects.
Industry: Utilized in the development of pharmaceuticals and nutraceuticals.
Comparison with Similar Compounds
(+/-)-Praeruptorin A can be compared with other coumarin derivatives such as:
Scopoletin: Known for its anti-inflammatory and antioxidant properties.
Umbelliferone: Exhibits similar anti-inflammatory and anti-cancer activities.
Aesculetin: Another coumarin with notable anti-inflammatory and anti-cancer effects.
What sets this compound apart is its unique combination of biological activities and its potential for therapeutic applications across multiple domains.
Properties
IUPAC Name |
[(9R,10S)-10-acetyloxy-8,8-dimethyl-2-oxo-9,10-dihydropyrano[2,3-f]chromen-9-yl] (Z)-2-methylbut-2-enoate |
Source
|
---|---|---|
Source | PubChem | |
URL | https://pubchem.ncbi.nlm.nih.gov | |
Description | Data deposited in or computed by PubChem | |
InChI |
InChI=1S/C21H22O7/c1-6-11(2)20(24)27-19-18(25-12(3)22)16-14(28-21(19,4)5)9-7-13-8-10-15(23)26-17(13)16/h6-10,18-19H,1-5H3/b11-6-/t18-,19+/m0/s1 |
Source
|
Source | PubChem | |
URL | https://pubchem.ncbi.nlm.nih.gov | |
Description | Data deposited in or computed by PubChem | |
InChI Key |
XGPBRZDOJDLKOT-CIRUDOSSSA-N |
Source
|
Source | PubChem | |
URL | https://pubchem.ncbi.nlm.nih.gov | |
Description | Data deposited in or computed by PubChem | |
Canonical SMILES |
CC=C(C)C(=O)OC1C(C2=C(C=CC3=C2OC(=O)C=C3)OC1(C)C)OC(=O)C |
Source
|
Source | PubChem | |
URL | https://pubchem.ncbi.nlm.nih.gov | |
Description | Data deposited in or computed by PubChem | |
Isomeric SMILES |
C/C=C(/C)\C(=O)O[C@@H]1[C@H](C2=C(C=CC3=C2OC(=O)C=C3)OC1(C)C)OC(=O)C |
Source
|
Source | PubChem | |
URL | https://pubchem.ncbi.nlm.nih.gov | |
Description | Data deposited in or computed by PubChem | |
Molecular Formula |
C21H22O7 |
Source
|
Source | PubChem | |
URL | https://pubchem.ncbi.nlm.nih.gov | |
Description | Data deposited in or computed by PubChem | |
Molecular Weight |
386.4 g/mol |
Source
|
Source | PubChem | |
URL | https://pubchem.ncbi.nlm.nih.gov | |
Description | Data deposited in or computed by PubChem | |
Disclaimer and Information on In-Vitro Research Products
Please be aware that all articles and product information presented on BenchChem are intended solely for informational purposes. The products available for purchase on BenchChem are specifically designed for in-vitro studies, which are conducted outside of living organisms. In-vitro studies, derived from the Latin term "in glass," involve experiments performed in controlled laboratory settings using cells or tissues. It is important to note that these products are not categorized as medicines or drugs, and they have not received approval from the FDA for the prevention, treatment, or cure of any medical condition, ailment, or disease. We must emphasize that any form of bodily introduction of these products into humans or animals is strictly prohibited by law. It is essential to adhere to these guidelines to ensure compliance with legal and ethical standards in research and experimentation.