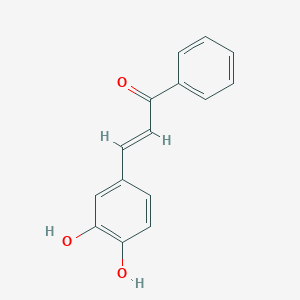
3,4-Dihydroxychalcone
- Click on QUICK INQUIRY to receive a quote from our team of experts.
- With the quality product at a COMPETITIVE price, you can focus more on your research.
Overview
Description
Antioxidant Agent-1 is a compound known for its ability to neutralize free radicals and prevent oxidative damage in various biological and industrial systems. Antioxidants are crucial in maintaining cellular health by mitigating the effects of oxidative stress, which can lead to chronic diseases and aging .
Preparation Methods
Synthetic Routes and Reaction Conditions: The synthesis of Antioxidant Agent-1 typically involves a series of organic reactions. One common method includes the condensation of specific aromatic aldehydes with phenolic compounds under acidic or basic conditions. The reaction is often catalyzed by acids like hydrochloric acid or bases like sodium hydroxide, followed by purification through recrystallization .
Industrial Production Methods: Industrial production of Antioxidant Agent-1 may involve large-scale chemical synthesis using continuous flow reactors. These reactors allow for precise control over reaction conditions, such as temperature and pressure, ensuring high yield and purity. The process may also include steps like solvent extraction and chromatography for purification .
Chemical Reactions Analysis
Types of Reactions: Antioxidant Agent-1 undergoes various chemical reactions, including:
Oxidation: It can be oxidized by reactive oxygen species, forming stable oxidation products.
Reduction: In the presence of reducing agents, it can revert to its original form.
Substitution: It can participate in electrophilic and nucleophilic substitution reactions, depending on the functional groups present.
Common Reagents and Conditions:
Oxidation: Hydrogen peroxide or potassium permanganate under acidic conditions.
Reduction: Sodium borohydride or lithium aluminum hydride in anhydrous solvents.
Substitution: Halogenating agents like chlorine or bromine in the presence of catalysts.
Major Products: The major products formed from these reactions depend on the specific conditions and reagents used. For example, oxidation typically yields quinones, while reduction can regenerate the original phenolic structure .
Scientific Research Applications
Antioxidant Agent-1 has a wide range of applications in scientific research:
Chemistry: Used as a standard antioxidant in various assays to study oxidative processes.
Biology: Investigated for its role in protecting cells from oxidative stress and its potential in anti-aging research.
Medicine: Explored for its therapeutic potential in treating diseases related to oxidative stress, such as cardiovascular diseases and neurodegenerative disorders.
Industry: Utilized in the food and cosmetic industries to prevent oxidation and extend shelf life.
Mechanism of Action
Antioxidant Agent-1 exerts its effects primarily through the neutralization of free radicals. It donates electrons to reactive oxygen species, converting them into less reactive molecules. This process involves several molecular targets and pathways, including:
Reactive Oxygen Species (ROS) Scavenging: Directly neutralizes ROS like superoxide anions and hydroxyl radicals.
Enzyme Modulation: Enhances the activity of endogenous antioxidant enzymes such as superoxide dismutase and catalase.
Signal Transduction Pathways: Modulates pathways like the Nrf2/ARE pathway, which regulates the expression of antioxidant proteins.
Comparison with Similar Compounds
Vitamin C (Ascorbic Acid): A well-known antioxidant that also scavenges free radicals and supports immune function.
Vitamin E (Tocopherol): Protects cell membranes from oxidative damage by reacting with lipid radicals.
Polyphenols: Found in plants, these compounds have multiple hydroxyl groups that contribute to their antioxidant activity.
Uniqueness: Antioxidant Agent-1 is unique due to its specific chemical structure, which allows it to participate in a broader range of reactions compared to other antioxidants. Its stability and reactivity make it particularly effective in various applications, from biological systems to industrial processes .
Properties
Molecular Formula |
C15H12O3 |
---|---|
Molecular Weight |
240.25 g/mol |
IUPAC Name |
(E)-3-(3,4-dihydroxyphenyl)-1-phenylprop-2-en-1-one |
InChI |
InChI=1S/C15H12O3/c16-13(12-4-2-1-3-5-12)8-6-11-7-9-14(17)15(18)10-11/h1-10,17-18H/b8-6+ |
InChI Key |
HHKVOYUYPYZFHJ-SOFGYWHQSA-N |
Isomeric SMILES |
C1=CC=C(C=C1)C(=O)/C=C/C2=CC(=C(C=C2)O)O |
Canonical SMILES |
C1=CC=C(C=C1)C(=O)C=CC2=CC(=C(C=C2)O)O |
Origin of Product |
United States |
Disclaimer and Information on In-Vitro Research Products
Please be aware that all articles and product information presented on BenchChem are intended solely for informational purposes. The products available for purchase on BenchChem are specifically designed for in-vitro studies, which are conducted outside of living organisms. In-vitro studies, derived from the Latin term "in glass," involve experiments performed in controlled laboratory settings using cells or tissues. It is important to note that these products are not categorized as medicines or drugs, and they have not received approval from the FDA for the prevention, treatment, or cure of any medical condition, ailment, or disease. We must emphasize that any form of bodily introduction of these products into humans or animals is strictly prohibited by law. It is essential to adhere to these guidelines to ensure compliance with legal and ethical standards in research and experimentation.