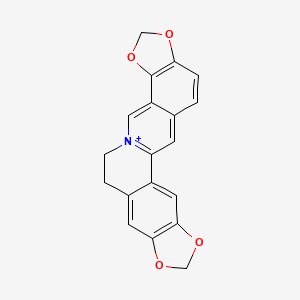
Coptisine
Overview
Description
Biochemical Analysis
Biochemical Properties
Coptisine interacts with various enzymes, proteins, and other biomolecules. It has been found to regulate signaling pathways such as NF-κB, MAPK, PI3K/Akt, NLRP3 inflammasome, RANKL/RANK, and Beclin 1/Sirt1 . The nature of these interactions involves the modulation of these pathways, contributing to its diverse beneficial properties .
Cellular Effects
This compound has been shown to have significant effects on various types of cells and cellular processes. It can inhibit the growth of gastric cancer cell lines ACC-201 and NCI-N87 in a dose-dependent manner . This compound also reduces inflammatory responses and improves glucose tolerance . It has been found to affect cell wall permeability and destroy cell structure .
Molecular Mechanism
This compound exerts its effects at the molecular level through various mechanisms. It has been found to interact with calf thymus DNA (ctDNA) through intercalation . It also has a neuroprotective effect on central nervous system diseases, particularly ischemic stroke . Furthermore, it has been shown to block the respiratory system by affecting the levels of critical enzymes of the tricarboxylic acid cycle and glycolysis .
Temporal Effects in Laboratory Settings
Over time, this compound has been observed to reduce cerebral infarct size and ameliorate neurological function score in cerebral ischemia-reperfusion (IR) rats . It also decreases inflammatory mediators such as prostaglandin D2 (PGD2) and tumor necrosis factor-α (TNF-α) after treatment .
Dosage Effects in Animal Models
The effects of this compound vary with different dosages in animal models. For instance, it has been observed that the plasma concentration of this compound demonstrates an obvious non-linear relationship with dosage . Even the highest dosage used in animal studies cannot reach the minimum concentration level used in cell experiments due to the poor absorption and low availability of this compound .
Metabolic Pathways
This compound is involved in multiple metabolic pathways. Dysregulations in fatty acids metabolism are associated with its neuroprotective and neuroinflammatory effects . It has also been found to regulate arachidonic acid metabolism .
Transport and Distribution
After intravenous administration, this compound quickly crosses the blood-brain barrier, accumulating at higher concentrations and then is slowly eliminated from different brain regions . This suggests that this compound can be effectively transported and distributed within cells and tissues.
Subcellular Localization
The subcellular localization of this compound and its effects on activity or function are not well defined in the literature. Given its ability to cross the blood-brain barrier and its interactions with various cellular components, it is likely that this compound may localize to specific compartments or organelles within the cell, which could influence its activity or function .
Preparation Methods
Synthetic Routes and Reaction Conditions: Coptisine can be synthesized through various chemical routes. One common method involves the extraction from the rhizomes of Coptis chinensis using solvents such as methanol or ethanol. The extract is then subjected to chromatographic techniques to isolate this compound .
Industrial Production Methods: In an industrial setting, this compound is typically produced through large-scale extraction processes. The rhizomes of Coptis chinensis are harvested, dried, and ground into a fine powder. This powder is then subjected to solvent extraction, followed by purification using techniques like high-performance liquid chromatography (HPLC) to obtain pure this compound .
Chemical Reactions Analysis
Types of Reactions: Coptisine undergoes various chemical reactions, including oxidation, reduction, and substitution reactions.
Common Reagents and Conditions:
Oxidation: this compound can be oxidized using reagents such as potassium permanganate or hydrogen peroxide under acidic conditions.
Reduction: Reduction of this compound can be achieved using reducing agents like sodium borohydride or lithium aluminum hydride.
Substitution: Substitution reactions involving this compound often use halogenating agents like bromine or chlorine under controlled conditions.
Major Products: The major products formed from these reactions depend on the specific reagents and conditions used. For example, oxidation of this compound can lead to the formation of this compound oxide, while reduction can yield this compound alcohol .
Scientific Research Applications
Mechanism of Action
Coptisine is often compared with other protoberberine alkaloids such as berberine, palmatine, and jatrorrhizine. While all these compounds share similar chemical structures, this compound is unique in its higher efficacy against certain cancer cell lines and its distinct molecular targets .
Comparison with Similar Compounds
Berberine: Known for its anti-diabetic and anti-inflammatory properties.
Palmatine: Exhibits neuroprotective and cardioprotective effects.
Jatrorrhizine: Has antimicrobial and anti-inflammatory activities.
Coptisine stands out due to its potent antibacterial and anti-cancer activities, making it a valuable compound for further research and therapeutic development.
Biological Activity
Coptisine, a prominent alkaloid derived from the roots of Coptis chinensis, has garnered significant attention in pharmacological research due to its diverse biological activities. This article provides a comprehensive overview of the biological activity of this compound, including its antibacterial, anti-inflammatory, and anticancer properties, supported by relevant case studies and research findings.
Chemical Structure and Properties
This compound is classified as a protoberberine alkaloid, characterized by its unique chemical structure which contributes to its biological activities. The molecular formula of this compound is C_20H_19NO_4, with a molecular weight of 339.37 g/mol. Its structure allows it to interact with various biological targets, making it a subject of interest in medicinal chemistry.
Antibacterial Activity
This compound exhibits potent antibacterial properties, particularly against Gram-negative bacteria such as Pasteurella multocida. Recent studies have demonstrated that this compound has a minimum inhibitory concentration (MIC) of 0.125 mg/mL , indicating strong antibacterial efficacy .
The antibacterial mechanism involves several pathways:
- Cell Wall Disruption : this compound alters cell wall permeability, leading to cell lysis.
- Inhibition of Respiratory Enzymes : It significantly reduces the activity of succinate dehydrogenase (SDH) and lactate dehydrogenase (LDH), crucial for bacterial respiration. For instance, SDH activity decreased from 31.53 ± 1.27 U/mg protein in untreated cells to 5.80 ± 0.74 U/mg protein in treated cells .
- Protein Synthesis Inhibition : this compound treatment resulted in a significant reduction in soluble protein synthesis within bacterial cells .
Anti-inflammatory Activity
This compound has shown promising anti-inflammatory effects in various animal models. In a study involving xylene-induced ear edema and carrageenan-induced paw edema models, this compound significantly reduced inflammation markers such as interleukin-1β (IL-1β) and tumor necrosis factor (TNF-α) .
Signaling Pathways
The anti-inflammatory action is mediated through inhibition of key signaling pathways:
- NF-κB Pathway : this compound inhibited the translocation of NF-κB components, thereby reducing inflammatory responses.
- MAPK Pathway : It also blocked the phosphorylation of p38 and JNK MAPK pathways, contributing to its anti-inflammatory effects .
Anticancer Activity
Emerging research indicates that this compound possesses anticancer properties. It has been shown to induce apoptosis in various cancer cell lines through mechanisms involving caspase activation and modulation of cell cycle regulators.
Case Studies
- Breast Cancer Cells : this compound treatment led to significant apoptosis in MCF-7 breast cancer cells, with increased caspase-3 activity observed.
- Liver Cancer Models : In hepatocellular carcinoma models, this compound demonstrated cytotoxic effects by inhibiting cell proliferation and inducing cell cycle arrest.
Comparative Biological Activities
A comparative analysis of this compound with other alkaloids from Coptis chinensis reveals its superior efficacy against Helicobacter pylori, highlighting its potential as a therapeutic agent for gastric disorders .
Biological Activity | This compound | Other Alkaloids |
---|---|---|
Antibacterial | Strong | Moderate |
Anti-inflammatory | High | Variable |
Anticancer | Significant | Low |
Properties
IUPAC Name |
5,7,17,19-tetraoxa-13-azoniahexacyclo[11.11.0.02,10.04,8.015,23.016,20]tetracosa-1(13),2,4(8),9,14,16(20),21,23-octaene | |
---|---|---|
Source | PubChem | |
URL | https://pubchem.ncbi.nlm.nih.gov | |
Description | Data deposited in or computed by PubChem | |
InChI |
InChI=1S/C19H14NO4/c1-2-16-19(24-10-21-16)14-8-20-4-3-12-6-17-18(23-9-22-17)7-13(12)15(20)5-11(1)14/h1-2,5-8H,3-4,9-10H2/q+1 | |
Source | PubChem | |
URL | https://pubchem.ncbi.nlm.nih.gov | |
Description | Data deposited in or computed by PubChem | |
InChI Key |
XYHOBCMEDLZUMP-UHFFFAOYSA-N | |
Source | PubChem | |
URL | https://pubchem.ncbi.nlm.nih.gov | |
Description | Data deposited in or computed by PubChem | |
Canonical SMILES |
C1C[N+]2=C(C=C3C=CC4=C(C3=C2)OCO4)C5=CC6=C(C=C51)OCO6 | |
Source | PubChem | |
URL | https://pubchem.ncbi.nlm.nih.gov | |
Description | Data deposited in or computed by PubChem | |
Molecular Formula |
C19H14NO4+ | |
Source | PubChem | |
URL | https://pubchem.ncbi.nlm.nih.gov | |
Description | Data deposited in or computed by PubChem | |
Related CAS |
6020-18-4 (chloride) | |
Record name | Coptisine | |
Source | ChemIDplus | |
URL | https://pubchem.ncbi.nlm.nih.gov/substance/?source=chemidplus&sourceid=0003486666 | |
Description | ChemIDplus is a free, web search system that provides access to the structure and nomenclature authority files used for the identification of chemical substances cited in National Library of Medicine (NLM) databases, including the TOXNET system. | |
DSSTOX Substance ID |
DTXSID10188404 | |
Record name | Coptisine | |
Source | EPA DSSTox | |
URL | https://comptox.epa.gov/dashboard/DTXSID10188404 | |
Description | DSSTox provides a high quality public chemistry resource for supporting improved predictive toxicology. | |
Molecular Weight |
320.3 g/mol | |
Source | PubChem | |
URL | https://pubchem.ncbi.nlm.nih.gov | |
Description | Data deposited in or computed by PubChem | |
CAS No. |
3486-66-6 | |
Record name | Coptisine | |
Source | CAS Common Chemistry | |
URL | https://commonchemistry.cas.org/detail?cas_rn=3486-66-6 | |
Description | CAS Common Chemistry is an open community resource for accessing chemical information. Nearly 500,000 chemical substances from CAS REGISTRY cover areas of community interest, including common and frequently regulated chemicals, and those relevant to high school and undergraduate chemistry classes. This chemical information, curated by our expert scientists, is provided in alignment with our mission as a division of the American Chemical Society. | |
Explanation | The data from CAS Common Chemistry is provided under a CC-BY-NC 4.0 license, unless otherwise stated. | |
Record name | Coptisine | |
Source | ChemIDplus | |
URL | https://pubchem.ncbi.nlm.nih.gov/substance/?source=chemidplus&sourceid=0003486666 | |
Description | ChemIDplus is a free, web search system that provides access to the structure and nomenclature authority files used for the identification of chemical substances cited in National Library of Medicine (NLM) databases, including the TOXNET system. | |
Record name | Coptisine | |
Source | EPA DSSTox | |
URL | https://comptox.epa.gov/dashboard/DTXSID10188404 | |
Description | DSSTox provides a high quality public chemistry resource for supporting improved predictive toxicology. | |
Record name | COPTISINE | |
Source | FDA Global Substance Registration System (GSRS) | |
URL | https://gsrs.ncats.nih.gov/ginas/app/beta/substances/0GCL71VN14 | |
Description | The FDA Global Substance Registration System (GSRS) enables the efficient and accurate exchange of information on what substances are in regulated products. Instead of relying on names, which vary across regulatory domains, countries, and regions, the GSRS knowledge base makes it possible for substances to be defined by standardized, scientific descriptions. | |
Explanation | Unless otherwise noted, the contents of the FDA website (www.fda.gov), both text and graphics, are not copyrighted. They are in the public domain and may be republished, reprinted and otherwise used freely by anyone without the need to obtain permission from FDA. Credit to the U.S. Food and Drug Administration as the source is appreciated but not required. | |
Record name | Coptisine | |
Source | Hazardous Substances Data Bank (HSDB) | |
URL | https://pubchem.ncbi.nlm.nih.gov/source/hsdb/8323 | |
Description | The Hazardous Substances Data Bank (HSDB) is a toxicology database that focuses on the toxicology of potentially hazardous chemicals. It provides information on human exposure, industrial hygiene, emergency handling procedures, environmental fate, regulatory requirements, nanomaterials, and related areas. The information in HSDB has been assessed by a Scientific Review Panel. | |
Q1: How does coptisine exert its anti-cancer effects?
A1: this compound exhibits anti-cancer activity through multiple mechanisms, including:
- Induction of Apoptosis: this compound triggers apoptosis in various cancer cell lines, such as hepatocellular carcinoma [, ], non-small cell lung cancer [], and colorectal cancer []. This apoptotic induction involves the activation of caspase cascades, upregulation of pro-apoptotic proteins (e.g., Bax), and downregulation of anti-apoptotic proteins (e.g., Bcl-2).
- Cell Cycle Arrest: this compound induces cell cycle arrest, primarily at the G2/M phase, by modulating cell cycle regulatory proteins like cyclin B1, cdc2, cdc25C, and p21 [, ].
- Inhibition of Metastasis: this compound suppresses cancer cell migration and invasion by downregulating matrix metalloproteinases (MMPs), particularly MMP-9, and upregulating tissue inhibitors of metalloproteinases (TIMPs), particularly TIMP-1 [].
- Modulation of Specific Signaling Pathways: this compound has been shown to influence several signaling pathways implicated in cancer development and progression, including PI3K/AKT [, , ], NF-κB [, , ], and MAPK [, , ] pathways.
Q2: Does this compound target specific receptors or enzymes?
A2: Research suggests this compound interacts with several molecular targets, including:
- 67-kDa Laminin Receptor (67LR): this compound binds to 67LR, leading to its activation and subsequent induction of apoptosis in human hepatoma cells. This interaction also influences the cyclic guanosine 3′,5′-monophosphate (cGMP) signaling pathway [].
- Urease: this compound demonstrates specific inhibition of Helicobacter pylori urease, both in vitro and in vivo, by targeting its active site sulfhydryl group and nickel metallocenter. Additionally, it interferes with urease maturation by inhibiting the activity of the accessory protein UreG [, ].
- Monoamine Oxidase (MAO): this compound competitively inhibits MAO activity, potentially impacting catecholamine levels at biologically active sites [].
Q3: What downstream effects are observed upon this compound treatment in models of inflammatory diseases?
A3: this compound exhibits anti-inflammatory effects by:
- Suppressing Inflammatory Cytokine Production: In various disease models, such as ulcerative colitis [], allergic rhinitis [], psoriasis [], and sepsis-induced lung injury [], this compound effectively reduces the levels of pro-inflammatory cytokines like TNF-α, IL-1β, IL-6, IL-17, and IL-22.
- Inhibiting NLRP3 Inflammasome Activation: this compound has been shown to repress the activation of the NLRP3 inflammasome, a key player in inflammatory responses. This inhibition results in decreased levels of inflammasome components and downstream inflammatory mediators [, ].
- Activating the Nrf2/HO-1 Signaling Pathway: this compound activates the Nrf2/HO-1 signaling pathway, known for its antioxidant and cytoprotective effects, thereby protecting against oxidative stress-induced damage in various cell types, including keratinocytes [].
Q4: What is the molecular formula and weight of this compound?
A4: this compound has the molecular formula C19H14NO4+ and a molecular weight of 336.33 g/mol (as the chloride salt) [].
Q5: Is there spectroscopic data available for this compound?
A5: Yes, researchers utilize various spectroscopic techniques to characterize this compound, including:
- Nuclear Magnetic Resonance (NMR): 1H-NMR, 13C-NMR, and 19F-NMR are used to confirm the structure of this compound and its derivatives, providing information about the arrangement of atoms and functional groups within the molecule [, , ].
- Ultraviolet-Visible (UV-Vis) Spectroscopy: UV-Vis spectroscopy is employed to study the complex formation between this compound and other molecules, such as β-cyclodextrin [].
- Mass Spectrometry (MS): High-resolution mass spectrometry (HRMS) is utilized for the accurate determination of the molecular weight and elemental composition of this compound and its derivatives [, ].
Q6: How do structural modifications of this compound influence its activity?
A6: * Dihydrocoptisines: Studies revealed that dihydrocoptisines, compared to quaternary coptisines and tetrahydrocoptisines, exhibit significantly higher anti-ulcerative colitis activity, attributed to their potent activation of XBP1 transcription [].* 8-Alkyl Substitution: Introducing alkyl chains at the 8-position of this compound can enhance its glucose-lowering effect in vitro. The length of the alkyl chain influences activity, with 8-hexyl-coptisine demonstrating the most significant effect on glucose consumption in HepG2 cells [].* Trifluoromethylation: Trifluoromethylation of dihydrocoptisines at the 8-position improves their structural stability and enhances their XBP1-activating activity, suggesting a potential strategy for developing more potent anti-ulcerative colitis agents [].
Q7: What is known about the absorption and bioavailability of this compound?
A7: this compound suffers from poor absorption in the gastrointestinal system, leading to low oral bioavailability. Studies in rats revealed that the absolute bioavailability of this compound ranged from 0.52% to 1.87% after oral administration []. This low bioavailability may hinder its clinical application, necessitating the development of strategies to enhance its absorption and systemic exposure.
Q8: What in vitro models have been used to study the effects of this compound?
A8: Various cell lines have been utilized to investigate the biological activities of this compound, including:
- Cancer Cell Lines: Human hepatoma cells (e.g., Hep3B, SMMC7721, HepG2) [, ], non-small cell lung cancer cells (e.g., A549, H460, H2170) [], colorectal cancer cells (e.g., HCT116) [], human breast cancer cells (e.g., MDA-MB-231) [], and human pancreatic cancer cells (e.g., PANC-1) [].
- Other Cell Types: Rat basophilic leukemia cells (RBL-2H3) [], human keratinocytes (HaCaT) [, ], murine lung epithelial cells (MLE-12) [], rat aortic rings [], mouse airway smooth muscle [], and rat articular chondrocytes [].
Q9: What in vivo models have been employed to evaluate this compound's therapeutic potential?
A9: Researchers have used a variety of animal models to assess this compound's efficacy, including:
- Rodent Models: Mouse models of Parkinson's disease [], ovalbumin-induced allergic rhinitis [], imiquimod-induced psoriasis [], pristane-induced lupus [], carbon tetrachloride-induced acute liver damage [], and diabetic nephropathy []. Rat models of orthodontic recurrence [], Helicobacter pylori infection [, ], post-infectious irritable bowel syndrome [], and L-NAME-induced hypertension [].
- Other Models: Zebrafish models have been used to assess the developmental toxicity of this compound [].
Q10: What is known about the stability of this compound?
A10: While specific stability data is limited in the provided research, this compound's quaternary ammonium structure suggests potential susceptibility to degradation pathways like hydrolysis.
Q11: Have any formulation strategies been explored to improve this compound's bioavailability?
A11: Yes, researchers have investigated formulation approaches to enhance this compound's solubility and bioavailability:
- β-Cyclodextrin Inclusion Complex: Formulating this compound hydrochloride with β-cyclodextrin significantly enhances its water solubility and stability, suggesting a promising strategy for improving its bioavailability [].
- Liposomal Nanoparticles: this compound encapsulated in liposomes has shown efficacy in a rat model of orthodontic recurrence, indicating the potential of nanocarriers for improving its delivery and therapeutic efficacy [].
Q12: What is the safety profile of this compound?
A12: While this compound exhibits promising pharmacological activities, it's crucial to acknowledge potential safety concerns:
- Hepatotoxicity: Although some studies suggest hepatoprotective effects of this compound [], other research indicates potential hepatotoxicity []. Thorough investigation of dose-dependent effects and long-term safety is essential.
- Developmental Toxicity: In zebrafish models, high doses of this compound caused morphological abnormalities in larvae, highlighting the importance of careful dose optimization and evaluation of potential developmental toxicity [].
Disclaimer and Information on In-Vitro Research Products
Please be aware that all articles and product information presented on BenchChem are intended solely for informational purposes. The products available for purchase on BenchChem are specifically designed for in-vitro studies, which are conducted outside of living organisms. In-vitro studies, derived from the Latin term "in glass," involve experiments performed in controlled laboratory settings using cells or tissues. It is important to note that these products are not categorized as medicines or drugs, and they have not received approval from the FDA for the prevention, treatment, or cure of any medical condition, ailment, or disease. We must emphasize that any form of bodily introduction of these products into humans or animals is strictly prohibited by law. It is essential to adhere to these guidelines to ensure compliance with legal and ethical standards in research and experimentation.