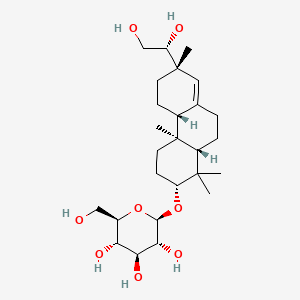
Darutoside
- Click on QUICK INQUIRY to receive a quote from our team of experts.
- With the quality product at a COMPETITIVE price, you can focus more on your research.
Overview
Description
Darutoside (Darutin) is a diterpenoid compound isolated from plants of the genus Siegesbeckia, notably S. glabrescens and S. orientalis . Structurally, it belongs to the ent-pimarane-type diterpenoids, with a molecular formula of C₂₆H₄₄O₈ and a molecular weight of 484.62 . This compound has demonstrated significant pharmacological activities, including:
- Anti-inflammatory effects: In rat models of acute gouty arthritis, this compound modulates serum metabolites (e.g., upregulating glutamate, alanine, and aspartic acid; downregulating uric acid, valine, and arachidonic acid) and influences pathways such as amino acid metabolism, purine metabolism, and arachidonic acid metabolism .
- Dermatological applications: It improves skin elasticity, collagen alignment, and wound healing, making it a component in topical formulations for stretch marks and anti-aging products .
- Antifertility activity: At doses of 20–40 mg/kg, this compound terminates early pregnancy in experimental rats .
Mechanistically, this compound regulates protein targets such as ALB, VEGFA, CASP3, and MAPK8, suggesting multi-target modulation in inflammatory and metabolic pathways .
Preparation Methods
Synthetic Routes and Reaction Conditions: Darutoside can be synthesized through various chemical processes. One method involves the extraction from the raw material of Siegesbeckia orientalis using ethanol. The process includes crushing the raw material, performing reflux extraction with ethanol, and then concentrating the extract . The concentrate is further processed using macroporous resin for adsorption, followed by elution with ethanol and reduced pressure concentration to obtain the final product .
Industrial Production Methods: The industrial production of this compound involves large-scale extraction techniques. The raw plant material is subjected to ethanol extraction, followed by purification using macroporous resin and high-speed counter-current chromatography . This method ensures high efficiency, large preparation capacity, and a relatively short production period .
Chemical Reactions Analysis
Types of Reactions: Darutoside undergoes various chemical reactions, including oxidation, reduction, and substitution. These reactions are essential for modifying its structure and enhancing its properties.
Common Reagents and Conditions:
Oxidation: Common oxidizing agents such as potassium permanganate or hydrogen peroxide can be used to oxidize this compound.
Reduction: Reducing agents like sodium borohydride or lithium aluminum hydride are employed to reduce this compound.
Substitution: Nucleophilic substitution reactions can be carried out using reagents like sodium hydroxide or potassium carbonate.
Major Products Formed: The major products formed from these reactions include various derivatives of this compound that possess enhanced biological activities and improved stability.
Scientific Research Applications
Darutoside has a wide range of scientific research applications, including:
Chemistry: It is used as a model compound for studying the chemical behavior of natural products and their derivatives.
Biology: this compound’s regenerative properties make it valuable in tissue engineering and regenerative medicine.
Medicine: It is widely used in dermatology for treating stretch marks, promoting wound healing, and reducing inflammation
Mechanism of Action
Darutoside exerts its effects through several molecular targets and pathways. It stimulates tissue regeneration by promoting the growth of the collagen matrix and enhancing skin microcirculation . Additionally, this compound regulates amino acid metabolism, sugar metabolism, fatty acid metabolism, and energy metabolism . It also inhibits oxidative stress and inflammatory responses, contributing to its anti-inflammatory properties .
Comparison with Similar Compounds
Structural and Functional Comparison with Kirenol
Kirenol, another diterpenoid from Siegesbeckia glabrescens, shares structural similarities with darutoside but exhibits distinct bioactivities:
Key Insight: While both compounds are anti-inflammatory, kirenol demonstrates stronger activity in classical inflammation models (e.g., LPS-induced cells), whereas this compound excels in metabolic modulation for gout .
Comparison with Neothis compound
Neothis compound , a structural analog isolated from S. glabrescens, differs in hydroxylation and glycosylation patterns:
- Structure: ent-3α,15,16-trihydroxy pimarane 3,15-bis-glucopyranoside .
Comparison with Triterpenoid Saponins (e.g., Ophiopogonin D′)
Ophiopogonin D′ (OPD′) is a triterpenoid saponin with contrasting structural class and bioactivity:
Key Insight : Unlike this compound, OPD′ targets apoptosis pathways in cancer, highlighting structural class-dependent divergence in therapeutic applications.
Comparison with Flavonoids (e.g., Rutin)
Rutin, a flavonoid co-occurring with this compound in Siegesbeckia extracts, differs mechanistically:
- Activity : Rutin exhibits antioxidant and anti-inflammatory effects via free radical scavenging, unlike this compound’s metabolic modulation .
- Synergy: In Siegesbeckia extracts, rutin and this compound may act complementarily, though this compound alone lacks NO suppression in RAW cells .
Discussion and Implications
- Structural determinants: Glycosylation in this compound enhances solubility and target engagement in metabolic pathways, whereas kirenol’s ent-kaurane skeleton favors NF-κB inhibition .
- Therapeutic niches: this compound is preferred for gout and dermatology, while kirenol and OPD′ are candidates for chronic inflammation and cancer, respectively .
Q & A
Basic Research Questions
Q. What experimental models are commonly used to evaluate Darutoside’s anti-inflammatory and metabolic regulatory effects?
Methodological Answer: this compound’s pharmacological activity is often assessed in rodent models of acute gouty arthritis, where inflammatory biomarkers (e.g., IL-1β, TNF-α) and metabolic pathways (amino acid, purine, and energy metabolism) are analyzed via liquid chromatography-mass spectrometry (LC-MS) and network pharmacology . In vitro studies use platelet aggregation assays under collagen stimulation to measure inhibitory effects, with U46619-induced rat platelet models being standard .
Q. How is this compound quantitatively analyzed in plant extracts or biological samples?
Methodological Answer: High-performance liquid chromatography (HPLC) with UV detection is validated for simultaneous quantification of this compound alongside structurally related diterpenoids (e.g., kirenol, darutigenol) in Siegesbeckia species. Key parameters include retention time (3.6–5.5 min), mobile phase optimization (acetonitrile/water gradients), and calibration curves with R² > 0.99 . For structural confirmation, 1D/2D NMR (COSY, HMBC) in solvents like DMSO-d6 or CD3OD provides definitive assignments of its ent-pimarane scaffold and glucoside moiety .
Q. What are the primary challenges in isolating this compound from natural sources?
Methodological Answer: this compound’s low abundance in Siegesbeckia spp. necessitates multi-step purification: (1) ethanol extraction, (2) silica gel chromatography with chloroform/methanol gradients, and (3) recrystallization. Co-elution with structurally similar compounds (e.g., darutigenol) requires countercurrent chromatography or preparative HPLC for resolution . Purity is confirmed via melting point analysis and HPLC-ELSD .
Advanced Research Questions
Q. How do molecular docking studies resolve contradictions in this compound’s dual inhibition of alanine aminotransferase (ALT) and aspartate aminotransferase (AST)?
Methodological Answer: Docking simulations (AutoDock Vina) reveal this compound binds to ALT and AST via conserved interactions: hydrogen bonds with Tyr216/Ser340 (ALT) and Trp140 (AST), and π-stacking with PLP cofactors. Despite similar binding energies (−139.84 kcal/mol for ALT vs. −153.75 kcal/mol for AST), MD simulations (>100 ns) show divergent stability in binding pockets, explaining its stronger ALT inhibition . Comparative mutagenesis studies (e.g., Tyr216Ala) validate these computational predictions .
Q. What multi-omics strategies are employed to elucidate this compound’s systemic effects on metabolic pathways?
Methodological Answer: Integrated metabolomics-pharmacology approaches identify 19 differential biomarkers (e.g., glutamate, chenodeoxycholic acid) in gouty arthritis models. LC-MS data are mapped to KEGG pathways (e.g., purine metabolism) and cross-validated with transcriptomics (RT-qPCR) of targets like VEGFA, CASP3, and STAT3. Network pharmacology (Cytoscape) further links this compound to hub proteins (ALB, HSP90AA1) via STRING database analysis .
Q. How can researchers address discrepancies in this compound’s reported solubility and bioavailability across studies?
Methodological Answer: Solubility variations arise from solvent polarity (e.g., 0.65 LogP in water vs. −2.08 in xanthurenic acid-β-glucoside) and crystallinity. Pre-formulation studies using differential scanning calorimetry (DSC) and particle size reduction (nanonization) improve bioavailability. Pharmacokinetic assays (LC-MS/MS) in Sprague-Dawley rats measure Cmax and T1/2, with enteric coatings mitigating first-pass metabolism .
Q. What experimental designs validate this compound’s synergistic effects with traditional Chinese medicine (TCM) formulations?
Methodological Answer: Isobolographic analysis quantifies synergy in TCM combinations (e.g., this compound + hesperidin). Fractional inhibitory concentration (FIC) indices < 0.5 indicate synergy in anti-inflammatory assays (LPS-induced RAW264.7 cells). Orthogonal partial least squares-discriminant analysis (OPLS-DA) of metabolomic data further identifies synergistic modulation of arachidonic acid pathways .
Q. Data Analysis & Validation
Q. How are conflicting results in this compound’s enzyme inhibition potency reconciled?
Methodological Answer: Discrepancies in IC50 values (e.g., ALT vs. AST) are addressed via (1) standardized assay conditions (pH 7.4, 37°C), (2) negative controls (PLP-free systems), and (3) surface plasmon resonance (SPR) to measure real-time binding kinetics. Meta-analysis of docking scores (ΔG) and experimental IC50 values using Pearson correlation (r > 0.85) ensures consistency .
Q. What statistical methods are critical for interpreting this compound’s multi-target effects in complex biological systems?
Methodological Answer: Multivariate analysis (PCA, OPLS-DA) reduces dimensionality in omics datasets, while false discovery rate (FDR) correction (Benjamini-Hochberg) minimizes Type I errors in pathway enrichment. Bayesian network modeling prioritizes high-confidence targets (e.g., MAPK8, PIK3CA) by integrating gene expression and metabolite flux data .
Q. Methodological Resources
Q. Where can researchers access validated protocols for this compound’s structural characterization?
Methodological Answer: The Beilstein Journal of Organic Chemistry provides guidelines for NMR data reporting (1H, 13C, HMBC in DMSO-d6/CD3OD) and purity verification (>95% via HPLC-ELSD). For novel derivatives, single-crystal X-ray diffraction (CCDC deposition) is recommended .
Properties
CAS No. |
59219-65-7 |
---|---|
Molecular Formula |
C26H44O8 |
Molecular Weight |
484.6 g/mol |
IUPAC Name |
(3R,4S,5S,6R)-2-[[(4aS,4bS,7S,10aS)-7-[(1R)-1,2-dihydroxyethyl]-1,4a,7,9-tetramethyl-1,2,3,4,4b,5,6,9,10,10a-decahydrophenanthren-2-yl]oxy]-6-(hydroxymethyl)oxane-3,4,5-triol |
InChI |
InChI=1S/C26H44O8/c1-13-9-17-14(2)18(33-24-23(32)22(31)21(30)19(11-27)34-24)6-8-26(17,4)16-5-7-25(3,10-15(13)16)20(29)12-28/h10,13-14,16-24,27-32H,5-9,11-12H2,1-4H3/t13?,14?,16-,17+,18?,19-,20+,21-,22+,23-,24?,25+,26-/m1/s1 |
InChI Key |
OJRJAOXVGPMJAP-XOQGPCJVSA-N |
Isomeric SMILES |
CC1C[C@H]2C(C(CC[C@@]2([C@H]3C1=C[C@@](CC3)(C)[C@H](CO)O)C)OC4[C@@H]([C@H]([C@@H]([C@H](O4)CO)O)O)O)C |
Canonical SMILES |
CC1CC2C(C(CCC2(C3C1=CC(CC3)(C)C(CO)O)C)OC4C(C(C(C(O4)CO)O)O)O)C |
Synonyms |
Darutin; Darutigenol 3-β-D-glucoside |
Origin of Product |
United States |
Disclaimer and Information on In-Vitro Research Products
Please be aware that all articles and product information presented on BenchChem are intended solely for informational purposes. The products available for purchase on BenchChem are specifically designed for in-vitro studies, which are conducted outside of living organisms. In-vitro studies, derived from the Latin term "in glass," involve experiments performed in controlled laboratory settings using cells or tissues. It is important to note that these products are not categorized as medicines or drugs, and they have not received approval from the FDA for the prevention, treatment, or cure of any medical condition, ailment, or disease. We must emphasize that any form of bodily introduction of these products into humans or animals is strictly prohibited by law. It is essential to adhere to these guidelines to ensure compliance with legal and ethical standards in research and experimentation.