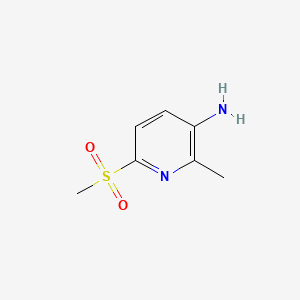
2-Methyl-6-(methylsulfonyl)pyridin-3-amine
Overview
Description
2-Methyl-6-(methylsulfonyl)pyridin-3-amine is a pyridine derivative characterized by a methyl group at position 2 and a methylsulfonyl group at position 6 on the pyridine ring. Its molecular formula is C₇H₁₀N₂O₂S, with a molecular weight of 186.23 g/mol (CAS: 897732-75-1) . Key synonyms include 3-Amino-2-methyl-6-(methylsulfonyl)pyridine and 6-(Methanesulfonyl)-2-methylpyridin-3-amine .
Preparation Methods
Synthetic Routes and Reaction Conditions
The synthesis of 2-Methyl-6-(methylsulfonyl)pyridin-3-amine can be achieved through several synthetic routes. One common method involves the Suzuki-Miyaura cross-coupling reaction. This reaction typically employs a palladium catalyst and boron reagents to form carbon-carbon bonds under mild and functional group-tolerant conditions . The starting materials for this synthesis include 5-bromo-2-methylpyridin-3-amine and methylsulfonyl chloride, which undergo coupling to yield the desired product.
Industrial Production Methods
Industrial production of this compound may involve large-scale Suzuki-Miyaura coupling reactions, optimized for high yield and purity. The reaction conditions, such as temperature, solvent, and catalyst concentration, are carefully controlled to ensure efficient production. Additionally, purification steps, such as recrystallization or chromatography, are employed to obtain the compound in its pure form.
Chemical Reactions Analysis
Types of Reactions
2-Methyl-6-(methylsulfonyl)pyridin-3-amine undergoes various chemical reactions, including:
Oxidation: The compound can be oxidized to form sulfone derivatives.
Reduction: Reduction reactions can convert the sulfonyl group to a sulfide.
Substitution: Nucleophilic substitution reactions can occur at the amine group or the pyridine ring.
Common Reagents and Conditions
Oxidation: Common oxidizing agents include hydrogen peroxide (H2O2) and potassium permanganate (KMnO4).
Reduction: Reducing agents such as lithium aluminum hydride (LiAlH4) or sodium borohydride (NaBH4) are used.
Substitution: Reagents like alkyl halides or acyl chlorides can be used for substitution reactions.
Major Products Formed
Oxidation: Sulfone derivatives.
Reduction: Sulfide derivatives.
Substitution: Various substituted pyridine derivatives, depending on the reagents used.
Scientific Research Applications
2-Methyl-6-(methylsulfonyl)pyridin-3-amine has several scientific research applications:
Chemistry: It is used as a building block in the synthesis of more complex organic molecules.
Biology: The compound is studied for its potential biological activities, including enzyme inhibition and receptor binding.
Medicine: Research is ongoing to explore its potential as a therapeutic agent for various diseases.
Industry: It is used in the development of new materials and chemical processes.
Mechanism of Action
The mechanism of action of 2-Methyl-6-(methylsulfonyl)pyridin-3-amine involves its interaction with specific molecular targets. For example, it may inhibit certain enzymes by binding to their active sites, thereby blocking their activity. The compound can also interact with receptors on cell surfaces, modulating signaling pathways and cellular responses .
Comparison with Similar Compounds
Comparison with Structurally Similar Compounds
Substituent Effects on Pyridine Derivatives
The following table compares 2-Methyl-6-(methylsulfonyl)pyridin-3-amine with pyridine-based analogs differing in substituents:
Key Observations:
- Electronic Effects : The methylsulfonyl group is strongly electron-withdrawing, reducing the basicity of the amine group compared to electron-donating substituents like pyrrolidinyl or methoxy .
- Polarity and Solubility: Sulfone-containing derivatives exhibit higher polarity, favoring solubility in polar solvents (e.g., DMSO, water) .
- Synthetic Accessibility : Sulfones are typically introduced via oxidation of sulfides or nucleophilic substitution, as seen in related imidazopyridine syntheses . Pyrrolidinyl and methoxy groups may require alkylation or coupling reactions .
Comparison with Sulfone-Containing Heterocycles
Imidazo[1,2-a]pyridine Derivatives
Compounds like 2-(ethylsulfinyl)imidazo[1,2-a]pyridine-3-sulfonamide (ZAPJAD) and 2-methyl-6-(trifluoromethyl)imidazo[1,2-a]pyridin-3-amine (ULEGOI) share sulfone/sulfonamide groups but differ in core structure :
- Core Structure : Imidazopyridines have fused aromatic systems, enhancing π-stacking interactions compared to simple pyridines.
- Biological Relevance : Sulfonamide imidazopyridines are explored for kinase inhibition, while pyridine sulfones may target receptors like serotonin or dopamine .
Aryl Sulfones in Medicinal Chemistry
In 2-(4-(methylsulfonyl)phenyl)-N-phenylimidazo[1,2-a]pyridin-3-amine derivatives, the methylsulfonyl group on a phenyl ring (vs. pyridine) improves metabolic stability and binding affinity in drug candidates . The direct attachment of sulfone to pyridine in the target compound may alter electronic effects and steric interactions.
Spectroscopic and Analytical Data
- IR Spectroscopy : Sulfone (SO₂) peaks appear at 1150–1300 cm⁻¹ , while NH stretches are observed at 3200–3450 cm⁻¹ . Pyrrolidinyl analogs show C-N stretches near 1200 cm⁻¹ .
- NMR : Methylsulfonyl protons resonate as a singlet at δ 3.2 ppm , while aromatic protons in pyridine appear at δ 7.9–8.3 ppm . Methoxy groups in analogs like 5-(2-Methoxypyridin-3-yl)pyridin-2-amine show signals at δ 3.8–4.0 ppm .
Biological Activity
2-Methyl-6-(methylsulfonyl)pyridin-3-amine, also known by its CAS number 897732-75-1, is a compound that has garnered interest in medicinal chemistry due to its potential biological activities. This article provides a comprehensive overview of the biological activity of this compound, focusing on its mechanisms, therapeutic applications, and relevant research findings.
Chemical Structure and Properties
The molecular structure of this compound features a pyridine ring substituted with a methyl group and a methylsulfonyl group. This unique arrangement contributes to its biological activity by influencing its interaction with various biological targets.
The biological activity of this compound is primarily attributed to its ability to modulate specific biochemical pathways. Research indicates that this compound can influence cAMP production in HEK293 cells, suggesting a role in signaling pathways that are crucial for various physiological processes . Additionally, it may interact with proteins involved in inflammatory responses and cancer progression.
Anticancer Activity
Recent studies have highlighted the anticancer potential of this compound. In vitro assays have demonstrated that this compound exhibits significant inhibitory effects on cancer cell lines. For instance, it has shown promising results against leukemia cell lines, where it induced apoptosis and inhibited cell proliferation .
Table 1: Anticancer Activity of this compound
Cell Line | IC50 (µM) | Mechanism of Action |
---|---|---|
MOLT-4 | 5.35 | Induction of apoptosis |
RPMI-8226 | 5.35 | Inhibition of cell proliferation |
T-47D | 5.35 | Cell cycle arrest in S-phase |
Anti-inflammatory Effects
The compound's anti-inflammatory properties have also been explored. It has been shown to inhibit COX enzymes, which play a crucial role in the inflammatory process. The IC50 values for COX inhibition suggest that it may be comparable to established anti-inflammatory drugs .
Table 2: Anti-inflammatory Activity
Enzyme | IC50 (µM) | Comparison Drug |
---|---|---|
COX-1 | 0.04 | Aspirin |
COX-2 | 0.04 | Celecoxib |
Case Studies and Research Findings
Several case studies have illustrated the potential therapeutic applications of this compound:
- Neurodegenerative Disorders : Inhibitors targeting neuronal nitric oxide synthase (nNOS) are critical for treating neurodegenerative diseases. Compounds structurally related to this pyridine derivative have been shown to selectively inhibit nNOS, suggesting potential applications in neuroprotection .
- Cancer Treatment : A study involving the treatment of various cancer cell lines revealed that derivatives similar to this compound significantly inhibited tumor growth and induced cell cycle arrest, particularly in breast and renal cancer models .
- Inflammatory Models : In vivo models demonstrated that treatment with this compound resulted in reduced inflammation markers and improved outcomes in carrageenan-induced paw edema tests, emphasizing its therapeutic potential in inflammatory diseases .
Q & A
Q. Basic: What synthetic methodologies are recommended for preparing 2-Methyl-6-(methylsulfonyl)pyridin-3-amine derivatives?
Answer:
The synthesis typically involves two-step reactions :
Intermediate Formation : React α-bromo-4-(methylsulfonyl)acetophenone with substituted anilines in methanol under basic conditions (e.g., NaHCO₃) to yield 1-(4-(methylsulfonyl)phenyl)-2-(phenylamino)ethan-1-one intermediates .
Cyclization : Condense intermediates with 2-aminopyridines in isopropanol at 80°C using ZnI₂ as a catalyst and 4 Å molecular sieves to form imidazo[1,2-a]pyridin-3-amine derivatives. Purification via recrystallization (ethanol) achieves >95% yield .
Key Validation : Monitor reaction completion via TLC and confirm purity using HPLC (>98%) .
Q. Advanced: How can structure-activity relationship (SAR) studies optimize COX-2 selectivity in these derivatives?
Answer:
SAR studies reveal that:
- Substituent Effects : Introducing a methyl group at the 8-position of the imidazo[1,2-a]pyridine core (e.g., compound 5n ) enhances COX-2 selectivity (IC₅₀ = 0.07 µM, selectivity index = 508.6) by fitting into the enzyme's hydrophobic pocket .
- Pharmacophore Alignment : The methylsulfonyl group forms hydrogen bonds with COX-2's secondary pocket (Arg513, His90), as shown in docking studies using AutoDock Vina .
Methodological Insight : Compare docking scores with reference drugs (e.g., celecoxib) and validate via in vitro COX-1/COX-2 inhibition assays .
Q. Basic: What spectroscopic techniques are critical for characterizing these compounds?
Answer:
- IR Spectroscopy : Confirm the presence of the methylsulfonyl group (SO₂) via peaks at 1150–1300 cm⁻¹ and NH stretches at 3200–3450 cm⁻¹ .
- NMR Analysis :
- LC-MS : Verify molecular ions (e.g., [M+H]⁺) and assess fragmentation patterns .
Q. Advanced: How do in vitro and in vivo assays correlate in evaluating pharmacological activity?
Answer:
- In Vitro Assays : COX-2 inhibition is quantified using enzyme immunoassays, measuring IC₅₀ values (e.g., 0.07–0.39 µM for derivatives). Selectivity indices (COX-1 IC₅₀/COX-2 IC₅₀) >100 indicate COX-2 specificity .
- In Vivo Validation : The formalin test evaluates antinociceptive activity. Compounds like 5r (N-(4-methoxyphenyl) derivative) reduce pain scores (AUC) by >50% in the inflammatory phase, correlating with COX-2 inhibition .
Data Interpretation : Compounds with high selectivity indices (e.g., 5n ) often show strong in vivo efficacy, but pharmacokinetic factors (e.g., solubility) require further optimization .
Q. Advanced: What computational strategies support the design of potent derivatives?
Answer:
- Molecular Docking : Use AutoDock Vina to position the methylsulfonyl pharmacophore into COX-2's secondary pocket. High-affinity poses (docking scores <−9.0 kcal/mol) predict potent inhibitors .
- Binding Mode Analysis : Hydrogen bonding with Arg513 and hydrophobic interactions with Val523 are critical. Compare with crystallographic ligands (e.g., SC-558) to validate docking accuracy .
Validation : Cross-check docking results with enzymatic assays. For example, 5n 's docking score (−10.2 kcal/mol) aligns with its submicromolar IC₅₀ .
Q. Methodological: How are intermediates and final compounds purified to ensure reproducibility?
Answer:
- Intermediate Purification : Filter crude products and wash with cold methanol/water to remove unreacted starting materials .
- Final Compound Recrystallization : Use ethanol or ethanol/water mixtures to achieve high purity (>98%). Monitor via HPLC (C18 column, acetonitrile/water mobile phase) .
Quality Control : Elemental analysis (C, H, N ±0.4%) ensures stoichiometric integrity .
Q. Advanced: What strategies mitigate synthetic challenges like byproduct formation?
Answer:
- Catalyst Optimization : ZnI₂ and molecular sieves enhance cyclization efficiency, reducing side products like unreacted intermediates .
- Reaction Monitoring : Use TLC (silica gel, ethyl acetate/hexane) to track reaction progress. Adjust reaction time (typically 8–12 hr) based on intermediate consumption .
Troubleshooting : If yields drop below 80%, re-optimize solvent (e.g., switch to anhydrous iPrOH) or catalyst loading .
Properties
IUPAC Name |
2-methyl-6-methylsulfonylpyridin-3-amine | |
---|---|---|
Source | PubChem | |
URL | https://pubchem.ncbi.nlm.nih.gov | |
Description | Data deposited in or computed by PubChem | |
InChI |
InChI=1S/C7H10N2O2S/c1-5-6(8)3-4-7(9-5)12(2,10)11/h3-4H,8H2,1-2H3 | |
Source | PubChem | |
URL | https://pubchem.ncbi.nlm.nih.gov | |
Description | Data deposited in or computed by PubChem | |
InChI Key |
UFMCZYUTXQRFKR-UHFFFAOYSA-N | |
Source | PubChem | |
URL | https://pubchem.ncbi.nlm.nih.gov | |
Description | Data deposited in or computed by PubChem | |
Canonical SMILES |
CC1=C(C=CC(=N1)S(=O)(=O)C)N | |
Source | PubChem | |
URL | https://pubchem.ncbi.nlm.nih.gov | |
Description | Data deposited in or computed by PubChem | |
Molecular Formula |
C7H10N2O2S | |
Source | PubChem | |
URL | https://pubchem.ncbi.nlm.nih.gov | |
Description | Data deposited in or computed by PubChem | |
DSSTOX Substance ID |
DTXSID60679615 | |
Record name | 6-(Methanesulfonyl)-2-methylpyridin-3-amine | |
Source | EPA DSSTox | |
URL | https://comptox.epa.gov/dashboard/DTXSID60679615 | |
Description | DSSTox provides a high quality public chemistry resource for supporting improved predictive toxicology. | |
Molecular Weight |
186.23 g/mol | |
Source | PubChem | |
URL | https://pubchem.ncbi.nlm.nih.gov | |
Description | Data deposited in or computed by PubChem | |
CAS No. |
897732-75-1 | |
Record name | 6-(Methanesulfonyl)-2-methylpyridin-3-amine | |
Source | EPA DSSTox | |
URL | https://comptox.epa.gov/dashboard/DTXSID60679615 | |
Description | DSSTox provides a high quality public chemistry resource for supporting improved predictive toxicology. | |
Synthesis routes and methods I
Procedure details
Synthesis routes and methods II
Procedure details
Synthesis routes and methods III
Procedure details
Disclaimer and Information on In-Vitro Research Products
Please be aware that all articles and product information presented on BenchChem are intended solely for informational purposes. The products available for purchase on BenchChem are specifically designed for in-vitro studies, which are conducted outside of living organisms. In-vitro studies, derived from the Latin term "in glass," involve experiments performed in controlled laboratory settings using cells or tissues. It is important to note that these products are not categorized as medicines or drugs, and they have not received approval from the FDA for the prevention, treatment, or cure of any medical condition, ailment, or disease. We must emphasize that any form of bodily introduction of these products into humans or animals is strictly prohibited by law. It is essential to adhere to these guidelines to ensure compliance with legal and ethical standards in research and experimentation.