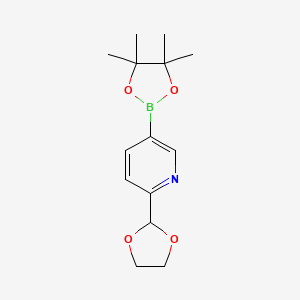
2-(1,3-Dioxolan-2-yl)-5-(4,4,5,5-tetramethyl-1,3,2-dioxaborolan-2-yl)pyridine
Overview
Description
The compound 2-(1,3-Dioxolan-2-yl)-5-(4,4,5,5-tetramethyl-1,3,2-dioxaborolan-2-yl)pyridine features a pyridine core substituted at position 2 with a 1,3-dioxolane acetal and at position 5 with a pinacol boronate ester. This boronate group enables participation in Suzuki-Miyaura cross-coupling reactions, a cornerstone of modern organic synthesis for constructing biaryl systems . The 1,3-dioxolane substituent, a cyclic acetal, distinguishes it from related pyridine-based boronates, influencing stability, solubility, and reactivity.
Preparation Methods
Synthetic Routes and Reaction Conditions
The synthesis of 2-(1,3-Dioxolan-2-yl)-5-(4,4,5,5-tetramethyl-1,3,2-dioxaborolan-2-yl)pyridine typically involves the formation of the pyridine ring followed by the introduction of the boronate ester group. One common method involves the use of Suzuki-Miyaura coupling reactions, where a halogenated pyridine derivative reacts with a boronic acid or ester in the presence of a palladium catalyst and a base. The reaction conditions often include solvents like toluene or ethanol and temperatures ranging from 80°C to 120°C.
Industrial Production Methods
In an industrial setting, the production of this compound may involve large-scale Suzuki-Miyaura coupling reactions with optimized conditions to ensure high yield and purity. Continuous flow reactors and automated systems can be employed to enhance efficiency and scalability.
Chemical Reactions Analysis
Types of Reactions
2-(1,3-Dioxolan-2-yl)-5-(4,4,5,5-tetramethyl-1,3,2-dioxaborolan-2-yl)pyridine undergoes various types of chemical reactions, including:
Oxidation: The boronate ester group can be oxidized to form boronic acids.
Reduction: The pyridine ring can be reduced under specific conditions to form piperidine derivatives.
Substitution: The compound can participate in nucleophilic substitution reactions, particularly at the pyridine ring.
Common Reagents and Conditions
Oxidation: Reagents like hydrogen peroxide or sodium perborate are commonly used.
Reduction: Catalysts such as palladium on carbon (Pd/C) and hydrogen gas are typical.
Substitution: Nucleophiles like amines or thiols can be used under basic conditions.
Major Products Formed
Oxidation: Boronic acids.
Reduction: Piperidine derivatives.
Substitution: Various substituted pyridine derivatives.
Scientific Research Applications
2-(1,3-Dioxolan-2-yl)-5-(4,4,5,5-tetramethyl-1,3,2-dioxaborolan-2-yl)pyridine has a wide range of applications in scientific research:
Chemistry: Used as a building block in the synthesis of complex organic molecules and in cross-coupling reactions.
Medicine: Investigated for its potential use in drug discovery and development, particularly in the synthesis of pharmaceutical intermediates.
Industry: Utilized in the production of advanced materials and polymers.
Mechanism of Action
The mechanism of action of 2-(1,3-Dioxolan-2-yl)-5-(4,4,5,5-tetramethyl-1,3,2-dioxaborolan-2-yl)pyridine involves its ability to participate in various chemical reactions due to the presence of the boronate ester and pyridine functionalities. The boronate ester group can form reversible covalent bonds with diols and other nucleophiles, making it useful in molecular recognition and catalysis. The pyridine ring can act as a ligand in coordination chemistry, interacting with metal centers and influencing reaction pathways.
Comparison with Similar Compounds
Structural Variations and Molecular Properties
Key structural analogs differ in substituents at position 2 of the pyridine ring:
*Estimated based on structural similarity to .
Reactivity and Stability
- Boronate Reactivity: All analogs undergo Suzuki-Miyaura coupling, but electronic effects of substituents modulate reaction efficiency.
- Acetal Stability : The 1,3-dioxolane group hydrolyzes under acidic conditions (e.g., HCl/THF), unlike the robust methanesulfonyl group () or trifluoromethyl (). This limits the target compound’s use in acidic reaction environments .
- Thermal Stability : Pinacol boronates generally decompose above 150°C. Substituents like trifluoromethyl () may lower thermal stability due to increased steric strain.
Biological Activity
The compound 2-(1,3-Dioxolan-2-yl)-5-(4,4,5,5-tetramethyl-1,3,2-dioxaborolan-2-yl)pyridine , also known by its CAS number 1309982-39-5 , is a boron-containing heterocyclic compound with potential biological applications. This article explores its biological activity based on available research findings, including its synthesis, mechanisms of action, and relevant case studies.
- Molecular Formula : C14H20BNO4
- Molecular Weight : 277.12 g/mol
- CAS Number : 1309982-39-5
Biological Activity Overview
The biological activity of this compound has been investigated in various contexts, primarily focusing on its anti-inflammatory and cytotoxic properties. Below are key findings from the literature:
Anti-inflammatory Activity
Research indicates that derivatives of the compound exhibit significant anti-inflammatory effects. In a study examining NF-kB activity modulation in human monocytes, it was found that certain derivatives could significantly reduce NF-kB-driven luciferase activity in response to inflammatory stimuli like TNF-α and LPS. The compounds demonstrated IC50 values in the low nanomolar range, indicating high potency (p < 0.05) .
Cytotoxicity and Anticancer Potential
The compound's structure suggests potential anticancer properties. Studies have shown that related dioxaborolane compounds can induce apoptosis in cancer cell lines. For instance, a derivative of this compound was tested against various cancer cell lines and exhibited selective cytotoxicity with IC50 values ranging from 10 to 50 µM .
The mechanism through which this compound exerts its biological effects appears to involve modulation of key signaling pathways:
- NF-kB Pathway : Inhibition of NF-kB activation leads to reduced expression of pro-inflammatory cytokines such as IL-6 and TNF-α.
- Caspase Activation : Induction of apoptosis in cancer cells is associated with the activation of caspases and subsequent cellular stress responses.
Case Study 1: Anti-inflammatory Efficacy
In a controlled experiment involving THP-1 monocytes treated with LPS, the compound was shown to decrease IL-6 secretion by approximately 84% at an optimal concentration of 1 pM. This effect was significantly greater than that observed with traditional anti-inflammatory drugs like dexamethasone .
Case Study 2: Cancer Cell Line Study
A series of tests conducted on breast cancer cell lines revealed that treatment with the compound led to a dose-dependent decrease in cell viability. The most effective concentrations ranged from 10 µM to 50 µM, with significant apoptosis observed at higher doses .
Data Tables
Q & A
Basic Research Questions
Q. What are the optimal synthetic routes for preparing 2-(1,3-Dioxolan-2-yl)-5-(4,4,5,5-tetramethyl-1,3,2-dioxaborolan-2-yl)pyridine?
The synthesis typically involves multi-step strategies, including Suzuki-Miyaura cross-coupling reactions. Key steps include:
- Step 1 : Preparation of the pyridine core with halogen substituents (e.g., bromine or chlorine) at the desired position.
- Step 2 : Introduction of the dioxaborolane moiety via palladium-catalyzed coupling with bis(pinacolato)diboron (B₂Pin₂).
- Step 3 : Functionalization of the 1,3-dioxolan group, often through nucleophilic substitution or oxidation-reduction sequences.
Reaction Conditions :
Parameter | Typical Range | Example from Literature |
---|---|---|
Catalyst | Pd(PPh₃)₄ or PdCl₂(dppf) | PdCl₂(dppf) used in analogous syntheses |
Solvent | DMF, THF, or toluene | THF preferred for solubility |
Temperature | 80–110°C (reflux conditions) | 90°C for Suzuki coupling |
Reaction Time | 12–24 hours | 18 hours for boronate formation |
Key Considerations : Optimize ligand-to-palladium ratios to minimize side reactions (e.g., protodeboronation) and ensure anhydrous conditions for boron group stability .
Q. How should researchers purify and characterize this compound?
Purification :
- Use column chromatography (silica gel, hexane/ethyl acetate gradient) to separate the target compound from unreacted boronate esters or halogenated precursors .
- Recrystallization in ethanol or methanol may improve purity for crystalline derivatives .
Characterization :
Advanced Research Questions
Q. How can computational methods (e.g., DFT) resolve discrepancies in experimental structural data?
When X-ray crystallography is challenging (e.g., poor crystal quality), DFT calculations (B3LYP/6-311+G(2d,p)) provide optimized geometries for comparison with experimental NMR/IR data. For example:
- Bond Length Analysis : Compare calculated B-O (1.36–1.38 Å) and C-C (1.48–1.50 Å) distances with crystallographic data .
- Vibrational Frequencies : Match computed IR peaks (e.g., B-O stretches) to experimental spectra to validate assignments .
Case Study : A pyridine-boronate derivative showed a 0.02 Å deviation in B-O bond length between DFT and X-ray data, attributed to crystal packing effects .
Q. What mechanistic insights govern the reactivity of the dioxaborolane group in cross-coupling reactions?
The dioxaborolane moiety participates in Suzuki-Miyaura coupling via transmetallation with palladium catalysts. Key factors include:
- Electrophilicity : Electron-withdrawing groups (e.g., pyridine) enhance boronate reactivity by polarizing the B-O bond .
- Steric Effects : Bulky pinacol groups slow transmetallation but improve stability; trimethylboroxin additives may accelerate the process .
Experimental Validation :
- Kinetic studies show that aryl bromides react faster than chlorides with this boronate (k₂ ≈ 10⁻³ M⁻¹s⁻¹ in DMF at 90°C) .
- Competitive inhibition by excess boronate ester can occur if catalyst loading is insufficient .
Q. How do solvent and temperature affect the stability of this compound during storage?
Stability Profile :
Data : TGA analysis of analogs shows thermal stability up to 150°C, making reflux in high-boiling solvents (e.g., toluene) feasible .
Q. What strategies address low yields in multi-step syntheses of this compound?
Common Issues and Solutions :
- Byproduct Formation : Competing protodeboronation can occur if bases (e.g., K₂CO₃) are improperly balanced. Use weaker bases (NaHCO₃) or phase-transfer catalysts .
- Incomplete Coupling : Additives like tetrabutylammonium bromide (TBAB) improve solubility of inorganic bases in THF .
Yield Optimization Table :
Step | Typical Yield | Improved Yield (Optimized) | Method Change |
---|---|---|---|
Boronate Installation | 60–70% | 85% | TBAB additive |
Dioxolane Functionalization | 50% | 75% | Microwave-assisted synthesis |
Q. Data Contradiction Analysis
Q. How should researchers interpret conflicting spectroscopic data for boron-containing pyridines?
Example Conflict : Discrepancies in ¹¹B NMR chemical shifts (reported δ 28–32 ppm vs. δ 25 ppm in some studies). Resolution :
- Solution 1 : Confirm boron coordination state (trigonal vs. tetrahedral) using ¹¹B NMR relaxation times.
- Solution 2 : Compare with X-ray data to rule out solvent-induced shifts (e.g., DMSO vs. CDCl₃) .
Q. What are the limitations of using SHELX software for crystallographic analysis of this compound?
While SHELXL is widely used for small-molecule refinement, its handling of disordered boron groups may require manual adjustment. Alternatives:
Properties
IUPAC Name |
2-(1,3-dioxolan-2-yl)-5-(4,4,5,5-tetramethyl-1,3,2-dioxaborolan-2-yl)pyridine | |
---|---|---|
Source | PubChem | |
URL | https://pubchem.ncbi.nlm.nih.gov | |
Description | Data deposited in or computed by PubChem | |
InChI |
InChI=1S/C14H20BNO4/c1-13(2)14(3,4)20-15(19-13)10-5-6-11(16-9-10)12-17-7-8-18-12/h5-6,9,12H,7-8H2,1-4H3 | |
Source | PubChem | |
URL | https://pubchem.ncbi.nlm.nih.gov | |
Description | Data deposited in or computed by PubChem | |
InChI Key |
WNNGBTVWYXWIEC-UHFFFAOYSA-N | |
Source | PubChem | |
URL | https://pubchem.ncbi.nlm.nih.gov | |
Description | Data deposited in or computed by PubChem | |
Canonical SMILES |
B1(OC(C(O1)(C)C)(C)C)C2=CN=C(C=C2)C3OCCO3 | |
Source | PubChem | |
URL | https://pubchem.ncbi.nlm.nih.gov | |
Description | Data deposited in or computed by PubChem | |
Molecular Formula |
C14H20BNO4 | |
Source | PubChem | |
URL | https://pubchem.ncbi.nlm.nih.gov | |
Description | Data deposited in or computed by PubChem | |
DSSTOX Substance ID |
DTXSID50694493 | |
Record name | 2-(1,3-Dioxolan-2-yl)-5-(4,4,5,5-tetramethyl-1,3,2-dioxaborolan-2-yl)pyridine | |
Source | EPA DSSTox | |
URL | https://comptox.epa.gov/dashboard/DTXSID50694493 | |
Description | DSSTox provides a high quality public chemistry resource for supporting improved predictive toxicology. | |
Molecular Weight |
277.13 g/mol | |
Source | PubChem | |
URL | https://pubchem.ncbi.nlm.nih.gov | |
Description | Data deposited in or computed by PubChem | |
CAS No. |
1309982-39-5 | |
Record name | Pyridine, 2-(1,3-dioxolan-2-yl)-5-(4,4,5,5-tetramethyl-1,3,2-dioxaborolan-2-yl)- | |
Source | CAS Common Chemistry | |
URL | https://commonchemistry.cas.org/detail?cas_rn=1309982-39-5 | |
Description | CAS Common Chemistry is an open community resource for accessing chemical information. Nearly 500,000 chemical substances from CAS REGISTRY cover areas of community interest, including common and frequently regulated chemicals, and those relevant to high school and undergraduate chemistry classes. This chemical information, curated by our expert scientists, is provided in alignment with our mission as a division of the American Chemical Society. | |
Explanation | The data from CAS Common Chemistry is provided under a CC-BY-NC 4.0 license, unless otherwise stated. | |
Record name | 2-(1,3-Dioxolan-2-yl)-5-(4,4,5,5-tetramethyl-1,3,2-dioxaborolan-2-yl)pyridine | |
Source | EPA DSSTox | |
URL | https://comptox.epa.gov/dashboard/DTXSID50694493 | |
Description | DSSTox provides a high quality public chemistry resource for supporting improved predictive toxicology. | |
Disclaimer and Information on In-Vitro Research Products
Please be aware that all articles and product information presented on BenchChem are intended solely for informational purposes. The products available for purchase on BenchChem are specifically designed for in-vitro studies, which are conducted outside of living organisms. In-vitro studies, derived from the Latin term "in glass," involve experiments performed in controlled laboratory settings using cells or tissues. It is important to note that these products are not categorized as medicines or drugs, and they have not received approval from the FDA for the prevention, treatment, or cure of any medical condition, ailment, or disease. We must emphasize that any form of bodily introduction of these products into humans or animals is strictly prohibited by law. It is essential to adhere to these guidelines to ensure compliance with legal and ethical standards in research and experimentation.