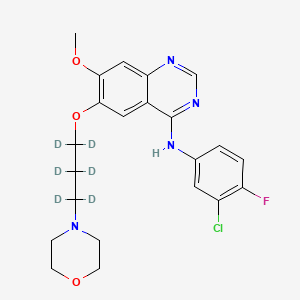
Gefitinib-d6
Overview
Description
Gefitinib-d6 is a deuterated form of gefitinib, a selective small-molecule epidermal growth factor receptor (EGFR) tyrosine kinase inhibitor. Gefitinib is primarily used for the treatment of non-small cell lung cancer (NSCLC) with activating mutations of EGFR tyrosine kinase . The deuterated version, this compound, is often used in scientific research to study the pharmacokinetics and metabolic pathways of gefitinib due to its enhanced stability and reduced metabolic rate .
Mechanism of Action
Target of Action
Gefitinib-d6, like its parent compound Gefitinib, primarily targets the Epidermal Growth Factor Receptor (EGFR) . EGFR is a tyrosine kinase that plays a crucial role in regulating cell proliferation, differentiation, and survival . It is often overexpressed in certain human carcinoma cells, such as lung and breast cancer cells .
Mode of Action
This compound acts as an inhibitor of the EGFR tyrosine kinase . It binds to the adenosine triphosphate (ATP)-binding site of the enzyme, thereby inhibiting the intracellular phosphorylation of numerous tyrosine kinases associated with transmembrane cell surface receptors . This selective inhibition disrupts the signaling cascades downstream of EGFR, leading to inhibited malignant cell proliferation .
Biochemical Pathways
The inhibition of EGFR by this compound affects several biochemical pathways. It perturbs signal transduction pathways, apoptosis, cell cycle, FOXO-mediated transcription, and the p53 signaling pathway . Notably, it has been observed that Gefitinib can regulate the negative interaction between MIG6 and STAT3 . This regulation plays a significant role in the sustained activation of STAT3 and resistance to EGFR-TKIs .
Pharmacokinetics
This compound, like Gefitinib, is extensively metabolized in patients . Excretion is predominantly via the feces, with renal elimination accounting for less than 4% of the administered dose . The ADME properties of this compound are expected to be similar to those of Gefitinib, which has a bioavailability of 59% and a protein binding of 90% .
Result of Action
The action of this compound leads to several molecular and cellular effects. It inhibits the proliferation of non-small cell lung cancer (NSCLC) cells and enhances apoptosis . It also inhibits migration in gefitinib-resistant lung cancer cell lines . At the molecular level, this compound causes a rapid increase in the level of the proapoptotic BH3-only protein BIM .
Action Environment
Environmental factors can influence the action, efficacy, and stability of this compound. For instance, the Predicted Environmental Concentration (PEC) and Predicted No Effect Concentration (PNEC) ratio suggest that the use of Gefitinib presents an insignificant risk to the environment . .
Biochemical Analysis
Biochemical Properties
Gefitinib-d6 plays a crucial role in biochemical reactions by inhibiting the activity of the epidermal growth factor receptor tyrosine kinase. This inhibition occurs through the binding of this compound to the adenosine triphosphate (ATP)-binding site of the enzyme, thereby preventing the phosphorylation of tyrosine residues on the receptor. This action blocks downstream signaling pathways that are essential for cell proliferation and survival. This compound interacts with various biomolecules, including the epidermal growth factor receptor, ATP, and other proteins involved in the EGFR signaling pathway .
Cellular Effects
This compound exerts significant effects on various types of cells, particularly those with overexpressed or mutated epidermal growth factor receptor. In cancer cells, this compound inhibits cell proliferation, induces apoptosis, and reduces cell migration and invasion. It influences cell signaling pathways by blocking the activation of downstream molecules such as phosphoinositide 3-kinase (PI3K), Akt, and extracellular signal-regulated kinase (ERK). Additionally, this compound affects gene expression by modulating the transcription of genes involved in cell cycle regulation, apoptosis, and metabolism .
Molecular Mechanism
The molecular mechanism of this compound involves its binding to the ATP-binding site of the epidermal growth factor receptor tyrosine kinase, leading to the inhibition of receptor autophosphorylation. This inhibition prevents the activation of downstream signaling cascades, including the PI3K/Akt and Ras/Raf/MEK/ERK pathways. By blocking these pathways, this compound effectively halts cell proliferation and induces apoptosis in cancer cells. Additionally, this compound can modulate gene expression by affecting transcription factors and other regulatory proteins .
Temporal Effects in Laboratory Settings
In laboratory settings, the effects of this compound can change over time due to factors such as stability, degradation, and cellular adaptation. This compound is generally stable under standard storage conditions, but its activity may decrease over extended periods or under suboptimal conditions. Long-term exposure to this compound in cell culture studies has shown sustained inhibition of cell proliferation and induction of apoptosis. Some cells may develop resistance to this compound over time, necessitating combination therapies or alternative treatment strategies .
Dosage Effects in Animal Models
The effects of this compound vary with different dosages in animal models. At lower doses, this compound effectively inhibits tumor growth and reduces tumor size in xenograft models of non-small cell lung cancer. At higher doses, this compound may cause toxic effects, including gastrointestinal disturbances, liver toxicity, and skin rashes. The therapeutic window for this compound is relatively narrow, and careful dose optimization is required to balance efficacy and safety .
Metabolic Pathways
This compound is metabolized primarily in the liver by cytochrome P450 enzymes, particularly CYP3A4. The metabolic pathways of this compound involve O-demethylation, oxidative defluorination, and oxidation of the quinazoline ring. These metabolic reactions result in the formation of various metabolites, some of which retain biological activity. The deuterium labeling in this compound allows for more accurate tracking of these metabolic processes in vivo and in vitro .
Transport and Distribution
This compound is transported and distributed within cells and tissues through various mechanisms. It is actively transported by ATP-binding cassette (ABC) transporters, such as ABCC10, which can influence its intracellular accumulation and efficacy. This compound is widely distributed in tissues, with higher concentrations observed in the liver, lungs, and tumors. The distribution of this compound is influenced by factors such as tissue perfusion, binding to plasma proteins, and cellular uptake mechanisms .
Subcellular Localization
This compound localizes to specific subcellular compartments, including the cytoplasm and cell membrane, where it interacts with the epidermal growth factor receptor. The subcellular localization of this compound is critical for its activity, as it needs to be in proximity to the receptor to exert its inhibitory effects. Additionally, this compound may undergo post-translational modifications that influence its localization and function within the cell .
Preparation Methods
Synthetic Routes and Reaction Conditions
The synthesis of Gefitinib-d6 involves the incorporation of deuterium atoms into the gefitinib molecule. One common method is the hydrogen-deuterium exchange reaction, where hydrogen atoms in the molecule are replaced with deuterium atoms using deuterated reagents under specific conditions . Another approach involves the use of deuterated starting materials in the synthesis of gefitinib, ensuring that the final product is deuterated .
Industrial Production Methods
Industrial production of this compound follows similar principles but on a larger scale. The process involves the use of high-purity deuterated reagents and solvents to ensure the consistency and quality of the final product. Advanced techniques such as high-performance liquid chromatography (HPLC) and mass spectrometry are employed to monitor the purity and isotopic composition of the product .
Chemical Reactions Analysis
Types of Reactions
Gefitinib-d6 undergoes various chemical reactions, including:
Oxidation: Involves the addition of oxygen or the removal of hydrogen. Common oxidizing agents include hydrogen peroxide and potassium permanganate.
Reduction: Involves the addition of hydrogen or the removal of oxygen. Common reducing agents include sodium borohydride and lithium aluminum hydride.
Substitution: Involves the replacement of one atom or group of atoms with another.
Common Reagents and Conditions
Oxidation: Hydrogen peroxide, potassium permanganate, and other oxidizing agents under acidic or basic conditions.
Reduction: Sodium borohydride, lithium aluminum hydride, and other reducing agents under controlled temperature and pressure.
Substitution: Halogens, nucleophiles, and other reagents under specific conditions such as temperature, pressure, and solvent choice.
Major Products Formed
The major products formed from these reactions depend on the specific conditions and reagents used. For example, oxidation of this compound can lead to the formation of hydroxylated or ketone derivatives, while reduction can yield deuterated alcohols or amines .
Scientific Research Applications
Gefitinib-d6 is widely used in scientific research for various applications:
Pharmacokinetics: Studying the absorption, distribution, metabolism, and excretion of gefitinib in the body.
Metabolic Pathways: Investigating the metabolic pathways and identifying metabolites of gefitinib.
Drug Development: Developing new drugs and formulations based on the pharmacokinetic and metabolic data obtained from this compound studies.
Cancer Research: Understanding the mechanisms of action and resistance of gefitinib in cancer cells
Comparison with Similar Compounds
Gefitinib-d6 is compared with other similar compounds such as erlotinib and afatinib:
Erlotinib: Another first-generation EGFR tyrosine kinase inhibitor with similar efficacy but different safety profiles.
Afatinib: A second-generation EGFR tyrosine kinase inhibitor that is more effective in certain cases but has a higher rate of adverse events compared to gefitinib.
This compound is unique due to its deuterated nature, which provides enhanced stability and reduced metabolic rate, making it a valuable tool in pharmacokinetic and metabolic studies .
Properties
IUPAC Name |
N-(3-chloro-4-fluorophenyl)-6-(1,1,2,2,3,3-hexadeuterio-3-morpholin-4-ylpropoxy)-7-methoxyquinazolin-4-amine | |
---|---|---|
Source | PubChem | |
URL | https://pubchem.ncbi.nlm.nih.gov | |
Description | Data deposited in or computed by PubChem | |
InChI |
InChI=1S/C22H24ClFN4O3/c1-29-20-13-19-16(12-21(20)31-8-2-5-28-6-9-30-10-7-28)22(26-14-25-19)27-15-3-4-18(24)17(23)11-15/h3-4,11-14H,2,5-10H2,1H3,(H,25,26,27)/i2D2,5D2,8D2 | |
Source | PubChem | |
URL | https://pubchem.ncbi.nlm.nih.gov | |
Description | Data deposited in or computed by PubChem | |
InChI Key |
XGALLCVXEZPNRQ-AQCDAZAYSA-N | |
Source | PubChem | |
URL | https://pubchem.ncbi.nlm.nih.gov | |
Description | Data deposited in or computed by PubChem | |
Canonical SMILES |
COC1=C(C=C2C(=C1)N=CN=C2NC3=CC(=C(C=C3)F)Cl)OCCCN4CCOCC4 | |
Source | PubChem | |
URL | https://pubchem.ncbi.nlm.nih.gov | |
Description | Data deposited in or computed by PubChem | |
Isomeric SMILES |
[2H]C([2H])(C([2H])([2H])N1CCOCC1)C([2H])([2H])OC2=C(C=C3C(=C2)C(=NC=N3)NC4=CC(=C(C=C4)F)Cl)OC | |
Source | PubChem | |
URL | https://pubchem.ncbi.nlm.nih.gov | |
Description | Data deposited in or computed by PubChem | |
Molecular Formula |
C22H24ClFN4O3 | |
Source | PubChem | |
URL | https://pubchem.ncbi.nlm.nih.gov | |
Description | Data deposited in or computed by PubChem | |
Molecular Weight |
452.9 g/mol | |
Source | PubChem | |
URL | https://pubchem.ncbi.nlm.nih.gov | |
Description | Data deposited in or computed by PubChem | |
Q1: The research mentions that Gefitinib enhances the inhibitory effect of Cisplatin when administered after Cisplatin treatment. What is the proposed mechanism behind this enhanced effect?
A1: While the research demonstrates the enhanced inhibitory effect of the Gefitinib and Cisplatin combination [, ], it doesn't delve into the specific mechanism behind this synergy. Further research is needed to understand if this enhanced effect is due to Gefitinib directly impacting Cisplatin's mechanism of action, influencing downstream pathways affected by Cisplatin, or a combination of both.
Q2: The study focuses on the in vivo effects of Gefitinib on a murine model. Were any in vitro studies conducted to assess Gefitinib's impact on H22 hepatocarcinoma cell proliferation or other cellular mechanisms?
A2: The provided abstracts [, ] don't mention any in vitro studies conducted on H22 hepatocarcinoma cells using Gefitinib. In vitro studies would be beneficial to elucidate the drug's direct effects on these cells, exploring mechanisms like cell cycle arrest, apoptosis induction, or alterations in signaling pathways.
Q3: The study focuses on a specific dosage and administration schedule for Gefitinib. Could altering the dosage or schedule further enhance its efficacy, potentially minimizing the required Cisplatin dose and reducing side effects?
A3: The research utilized a specific Gefitinib dosage and schedule (100 mg/kg body weight daily) [, ]. Whether modifying this regimen could yield improved efficacy or allow for Cisplatin dose reduction requires further investigation. Exploring different dosages and schedules, alongside in vitro studies to determine optimal drug exposure, could provide valuable insights into maximizing therapeutic benefit while minimizing potential side effects.
Disclaimer and Information on In-Vitro Research Products
Please be aware that all articles and product information presented on BenchChem are intended solely for informational purposes. The products available for purchase on BenchChem are specifically designed for in-vitro studies, which are conducted outside of living organisms. In-vitro studies, derived from the Latin term "in glass," involve experiments performed in controlled laboratory settings using cells or tissues. It is important to note that these products are not categorized as medicines or drugs, and they have not received approval from the FDA for the prevention, treatment, or cure of any medical condition, ailment, or disease. We must emphasize that any form of bodily introduction of these products into humans or animals is strictly prohibited by law. It is essential to adhere to these guidelines to ensure compliance with legal and ethical standards in research and experimentation.