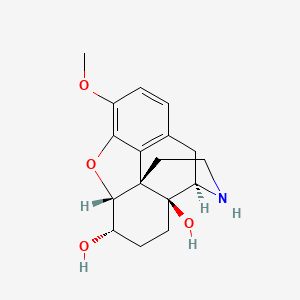
nor-6alpha-Oxycodol
Overview
Description
Nor-6alpha-Oxycodol is a metabolite of oxycodone, an opioid analgesic commonly used for pain management. This compound is structurally similar to known opioids and is primarily used as an analytical reference material in research and forensic applications .
Mechanism of Action
Target of Action
Nor-6alpha-Oxycodol, also known as alpha-noroxycodol, is a metabolite of oxycodone . It is structurally similar to known opioids . The primary targets of this compound are likely to be the same as those of oxycodone, which primarily targets the μ-opioid receptors .
Mode of Action
As a μ-opioid receptor agonist, this compound is expected to bind to these receptors, leading to a decrease in the perception of pain
Biochemical Pathways
Oxycodone has been shown to cause an up-regulation in GABA B receptor expression in sensory neurons . These receptors are transported to their central terminals within the dorsal horn, reinforcing a presynaptic inhibition .
Pharmacokinetics
It is known that this compound is a metabolite of oxycodone . Oxycodone is primarily metabolized via cytochrome P450 (CYP) 3A-mediated N-demethylation . The metabolites derived from this pathway, including this compound, account for 45% of the dose .
Result of Action
Given its structural similarity to known opioids, it is likely to have similar effects, such as analgesia .
Action Environment
The action, efficacy, and stability of this compound can be influenced by various environmental factors. These may include the presence of other drugs, the patient’s metabolic state, and genetic factors affecting drug metabolism. For instance, the presence of CYP3A inducers or inhibitors can substantially affect the pharmacokinetics and pharmacodynamics of oxycodone , and likely also its metabolites, including this compound.
Biochemical Analysis
Biochemical Properties
Alpha-Noroxycodol is a product of the metabolic pathway of oxycodone, which is primarily mediated by cytochrome P450 (CYP) 3A It interacts with various enzymes and proteins in the body
Cellular Effects
It is known that the central opioid effects of oxycodone, from which Alpha-Noroxycodol is derived, are governed by the parent drug, with a negligible contribution from its circulating oxidative and reductive metabolites .
Molecular Mechanism
It is known that oxycodone, the parent drug, interacts predominantly with the opioid mu-receptor . These mu-binding sites are discretely distributed in the human brain, with high densities in the posterior amygdala, hypothalamus, thalamus, nucleus caudatus, putamen, and certain cortical areas .
Metabolic Pathways
Alpha-Noroxycodol is a product of the metabolic pathway of oxycodone, which is primarily mediated by cytochrome P450 (CYP) 3A
Preparation Methods
Synthetic Routes and Reaction Conditions: The synthesis of nor-6alpha-Oxycodol involves the N-demethylation of oxycodone. This process is typically carried out in the liver via the enzyme cytochrome P450 3A4 (CYP3A4) . The reaction conditions for laboratory synthesis often involve the use of specific solvents such as dimethylformamide (DMF) and dimethyl sulfoxide (DMSO), with solubility in DMF being 20 mg/ml and in DMSO being 10 mg/ml .
Industrial Production Methods: Industrial production methods for this compound are not well-documented in the public domain. it is likely that large-scale synthesis would follow similar pathways as laboratory synthesis, with optimizations for yield and purity.
Chemical Reactions Analysis
Types of Reactions: Nor-6alpha-Oxycodol undergoes several types of chemical reactions, including:
Oxidation: This reaction can be facilitated by oxidizing agents such as hydrogen peroxide or potassium permanganate.
Reduction: Reduction reactions may involve reagents like lithium aluminum hydride.
Substitution: Substitution reactions can occur with halogenating agents or other nucleophiles.
Common Reagents and Conditions:
Oxidation: Hydrogen peroxide in an acidic medium.
Reduction: Lithium aluminum hydride in anhydrous ether.
Substitution: Halogenating agents like thionyl chloride.
Major Products: The major products formed from these reactions depend on the specific conditions and reagents used. For example, oxidation may yield nor-6alpha-Oxycodone N-oxide, while reduction could produce a deoxygenated derivative.
Scientific Research Applications
Nor-6alpha-Oxycodol is primarily used in scientific research as an analytical reference material. Its applications include:
Chemistry: Used in the study of opioid metabolism and the identification of new metabolites.
Biology: Employed in research on opioid receptor binding and activity.
Medicine: Investigated for its potential analgesic properties and its role in pain management.
Industry: Utilized in forensic toxicology for the identification and quantification of oxycodone metabolites.
Comparison with Similar Compounds
Noroxycodone: Another metabolite of oxycodone with similar properties but higher central nervous system penetration.
Noroxymorphone: A potent opioid with higher affinity for the mu-opioid receptor.
Norbuprenorphine: A metabolite of buprenorphine with distinct pharmacological properties.
Uniqueness: Nor-6alpha-Oxycodol is unique due to its specific structural configuration and its role as a minor metabolite of oxycodone. Its poor blood-brain barrier penetration differentiates it from other similar compounds, making it less effective as an analgesic but valuable for research purposes .
Properties
IUPAC Name |
(4R,4aS,7S,7aR,12bS)-9-methoxy-2,3,4,5,6,7,7a,13-octahydro-1H-4,12-methanobenzofuro[3,2-e]isoquinoline-4a,7-diol | |
---|---|---|
Source | PubChem | |
URL | https://pubchem.ncbi.nlm.nih.gov | |
Description | Data deposited in or computed by PubChem | |
InChI |
InChI=1S/C17H21NO4/c1-21-11-3-2-9-8-12-17(20)5-4-10(19)15-16(17,6-7-18-12)13(9)14(11)22-15/h2-3,10,12,15,18-20H,4-8H2,1H3/t10-,12+,15-,16-,17+/m0/s1 | |
Source | PubChem | |
URL | https://pubchem.ncbi.nlm.nih.gov | |
Description | Data deposited in or computed by PubChem | |
InChI Key |
KFWOOLJUSYSBAD-NZQXGWJPSA-N | |
Source | PubChem | |
URL | https://pubchem.ncbi.nlm.nih.gov | |
Description | Data deposited in or computed by PubChem | |
Canonical SMILES |
COC1=C2C3=C(CC4C5(C3(CCN4)C(O2)C(CC5)O)O)C=C1 | |
Source | PubChem | |
URL | https://pubchem.ncbi.nlm.nih.gov | |
Description | Data deposited in or computed by PubChem | |
Isomeric SMILES |
COC1=C2C3=C(C[C@@H]4[C@]5([C@]3(CCN4)[C@@H](O2)[C@H](CC5)O)O)C=C1 | |
Source | PubChem | |
URL | https://pubchem.ncbi.nlm.nih.gov | |
Description | Data deposited in or computed by PubChem | |
Molecular Formula |
C17H21NO4 | |
Source | PubChem | |
URL | https://pubchem.ncbi.nlm.nih.gov | |
Description | Data deposited in or computed by PubChem | |
DSSTOX Substance ID |
DTXSID801344992 | |
Record name | nor-6alpha-Oxycodol | |
Source | EPA DSSTox | |
URL | https://comptox.epa.gov/dashboard/DTXSID801344992 | |
Description | DSSTox provides a high quality public chemistry resource for supporting improved predictive toxicology. | |
Molecular Weight |
303.35 g/mol | |
Source | PubChem | |
URL | https://pubchem.ncbi.nlm.nih.gov | |
Description | Data deposited in or computed by PubChem | |
CAS No. |
116499-16-2 | |
Record name | nor-6alpha-Oxycodol | |
Source | EPA DSSTox | |
URL | https://comptox.epa.gov/dashboard/DTXSID801344992 | |
Description | DSSTox provides a high quality public chemistry resource for supporting improved predictive toxicology. | |
Retrosynthesis Analysis
AI-Powered Synthesis Planning: Our tool employs the Template_relevance Pistachio, Template_relevance Bkms_metabolic, Template_relevance Pistachio_ringbreaker, Template_relevance Reaxys, Template_relevance Reaxys_biocatalysis model, leveraging a vast database of chemical reactions to predict feasible synthetic routes.
One-Step Synthesis Focus: Specifically designed for one-step synthesis, it provides concise and direct routes for your target compounds, streamlining the synthesis process.
Accurate Predictions: Utilizing the extensive PISTACHIO, BKMS_METABOLIC, PISTACHIO_RINGBREAKER, REAXYS, REAXYS_BIOCATALYSIS database, our tool offers high-accuracy predictions, reflecting the latest in chemical research and data.
Strategy Settings
Precursor scoring | Relevance Heuristic |
---|---|
Min. plausibility | 0.01 |
Model | Template_relevance |
Template Set | Pistachio/Bkms_metabolic/Pistachio_ringbreaker/Reaxys/Reaxys_biocatalysis |
Top-N result to add to graph | 6 |
Feasible Synthetic Routes
Disclaimer and Information on In-Vitro Research Products
Please be aware that all articles and product information presented on BenchChem are intended solely for informational purposes. The products available for purchase on BenchChem are specifically designed for in-vitro studies, which are conducted outside of living organisms. In-vitro studies, derived from the Latin term "in glass," involve experiments performed in controlled laboratory settings using cells or tissues. It is important to note that these products are not categorized as medicines or drugs, and they have not received approval from the FDA for the prevention, treatment, or cure of any medical condition, ailment, or disease. We must emphasize that any form of bodily introduction of these products into humans or animals is strictly prohibited by law. It is essential to adhere to these guidelines to ensure compliance with legal and ethical standards in research and experimentation.