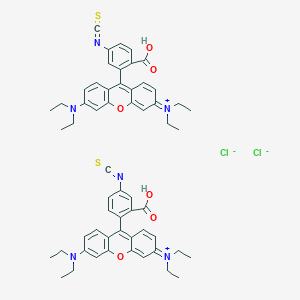
Rhodamine B isothiocyanate (mixed isomers)
Overview
Description
Rhodamine B isothiocyanate (RBITC) is a fluorescent dye derived from rhodamine B, featuring an isothiocyanate (-N=C=S) reactive group. This group enables covalent conjugation to primary amines (e.g., lysine residues) in proteins, forming stable thiourea bonds . RBITC exhibits excitation/emission maxima near 560 nm/580 nm, making it suitable for red fluorescence channels in microscopy and flow cytometry . It is widely used in biomedical research for labeling antibodies, tracking protein dynamics, and studying vascular permeability . RBITC’s mixed isomers (structural variants) provide flexibility in conjugation efficiency under alkaline conditions (pH 9.3) .
Mechanism of Action
Target of Action
Rhodamine B Isothiocyanate (RBITC) is primarily used as a fluorogenic dye . It is often conjugated to antibodies and proteins, serving as a fluorescent tag for fluorescence detection in microscopy . The primary targets of RBITC are therefore the proteins or antibodies to which it is conjugated.
Mode of Action
RBITC interacts with its targets (proteins or antibodies) through covalent attachment. The isothiocyanate functional group in RBITC forms a stable thiourea linkage with amino groups on proteins and other biomolecules . This interaction results in the protein or antibody being fluorescently labeled, allowing for detection and visualization in microscopy applications .
Biochemical Pathways
The exact biochemical pathways affected by RBITC depend on the specific proteins or antibodies to which it is conjugated. Instead, it serves as a tool for visualizing and tracking the behavior of its target molecules within these pathways .
Pharmacokinetics
Its bioavailability would be determined by factors such as its degree of conjugation to target molecules and the specific conditions of the experimental system .
Result of Action
The primary result of RBITC’s action is the fluorescent labeling of target proteins or antibodies. This allows for the visualization of these targets under a microscope, aiding in various biological research applications . For example, it can be used to track the movement of these molecules within cells, or to identify the presence of specific antigens in immunohistochemistry .
Action Environment
The action of RBITC can be influenced by various environmental factors. For instance, the fluorescence of RBITC is dependent on the pH of the environment . Additionally, the stability of the RBITC conjugate can be affected by temperature, with storage typically recommended at −20°C . The solvent used can also impact the fluorescence properties of RBITC .
Biochemical Analysis
Biochemical Properties
Rhodamine B isothiocyanate (mixed isomers) is commonly used as a conjugate to antibodies and proteins for fluorescence detection in microscopy . It exhibits not only a strong surface-enhanced Raman scattering (SERS) signal but also a measurable amount of fluorescence . The Rhodamine B isothiocyanate (mixed isomers)-modified Ag-deposited silica or polystyrene beads disperse well in ethanol . They are also readily coated in water with polyelectrolytes for their further derivatization with biological molecules of interest that can bind to target molecules .
Cellular Effects
Rhodamine B isothiocyanate (mixed isomers) is a cell-permeant fluorogenic dye . It can be used in conjunction with immunohistochemistry for multi-labeling studies . It has also been used as an anterograde and retrograde tracer in neurons and in nanoparticles to detect apoptosis .
Molecular Mechanism
It is known that it can bind to various biomolecules, including proteins and antibodies, which allows it to be used as a fluorescent tag . This binding can influence the function of these biomolecules, potentially leading to changes in cellular processes.
Temporal Effects in Laboratory Settings
It is known that Rhodamine B isothiocyanate (mixed isomers) exhibits a strong fluorescence signal, which can be used to track the movement and interactions of tagged biomolecules over time .
Biological Activity
Rhodamine B isothiocyanate (RBITC), a fluorescent dye, is widely utilized in biological applications due to its cell-permeant properties and ability to serve as a molecular tool in various biochemical assays. This article explores the biological activity of RBITC, focusing on its applications in cellular imaging, drug delivery systems, and its role in apoptosis detection.
- Molecular Formula : C_21H_18ClN_3O_5S
- Molecular Weight : 536.1 g/mol
- Structure : RBITC is characterized by its isothiocyanate functional group which enhances its reactivity and binding capabilities with biomolecules.
1. Cellular Imaging
RBITC is extensively used as a fluorescent tag for various biological molecules. It has been employed in studies to visualize cellular processes, including endocytosis and apoptosis. The dye’s fluorescence allows for real-time tracking of cellular activities.
Table 1: Applications of RBITC in Cellular Imaging
2. Drug Delivery Systems
Recent studies have demonstrated the use of RBITC in drug delivery systems, particularly in targeted cancer therapies. RBITC-modified nanoparticles have shown promise in enhancing the specificity and efficacy of drug delivery.
Case Study: Doxorubicin-Loaded Nanoparticles
In a study involving doxorubicin-loaded magnetic silica nanoparticles (Dox-MagSiNs) conjugated with RBITC, researchers observed effective targeting and release of the drug in cancer cells while minimizing toxicity to normal cells. The results indicated over 50% cell death in prostate and ovarian cancer cells, showcasing the potential of RBITC in improving therapeutic outcomes.
Table 2: Efficacy of Dox-MagSiNs Conjugated with RBITC
Cell Line | % Cell Death (with Dox-MagSiNs) | % Cell Death (without Dox-MagSiNs) |
---|---|---|
PC3 (Prostate) | >50% | <10% |
A2780 (Ovarian) | >50% | <15% |
MDAMB231 (Breast) | >125% improvement | - |
RBITC's mechanism involves its interaction with cellular components through covalent bonding, particularly with thiol groups present in proteins. This interaction facilitates the dye's uptake into cells via endocytosis, allowing for effective labeling and tracking.
Cytotoxicity and Biocompatibility
While RBITC is generally regarded as biocompatible, it exhibits cytotoxic effects at higher concentrations. Studies have shown that when used within recommended limits, RBITC does not significantly affect cell viability. However, caution is advised due to its classification as a potential irritant.
Table 3: Cytotoxicity Profile of RBITC
Concentration (µM) | Cell Viability (%) |
---|---|
1 | 95 |
10 | 85 |
50 | 60 |
Q & A
Basic Research Questions
Q. How do I conjugate Rhodamine B isothiocyanate (RBITC) to proteins or antibodies for fluorescence labeling?
RBITC reacts covalently with primary amines (e.g., lysine residues) in proteins under alkaline conditions (pH 8.5–9.5). A standard protocol involves:
- Dissolving RBITC in anhydrous DMSO or DMF to prepare a 10 mg/mL stock solution .
- Incubating the protein solution (1–2 mg/mL in 0.1 M carbonate buffer, pH 9.0) with RBITC at a 10:1 molar ratio (dye:protein) for 2 hours at 4°C in the dark .
- Removing unbound dye via gel filtration chromatography or dialysis .
- Validating conjugation efficiency using UV-Vis spectroscopy (RBITC absorbance at 570 nm) or fluorescence microscopy .
Q. What are the optimal storage conditions for RBITC to maintain fluorescence stability?
- Store lyophilized RBITC powder at -20°C in a desiccator protected from light and moisture.
- Prepare stock solutions in DMSO or DMF, aliquot, and store at -80°C for up to 6 months or -20°C for 1 month. Avoid repeated freeze-thaw cycles .
Q. Which solvents are compatible with RBITC for preparing working solutions?
RBITC is soluble in DMF (12.5 mg/mL), DMSO , ethanol , and PBS (with sonication). For in vivo studies, use formulations like 20% SBE-β-CD in saline or PEG400 to enhance solubility .
Q. What are the excitation/emission maxima of RBITC, and how do they compare to other rhodamine derivatives?
RBITC has excitation/emission maxima at 570/595 nm , making it suitable for red-channel imaging. Unlike fluorescein (green fluorescence) or tetramethylrhodamine (higher brightness), RBITC offers superior tumor-selective activation and photostability in acidic microenvironments .
Q. What is the basic protocol for neuronal tracing using RBITC?
RBITC is used as an anterograde/retrograde tracer due to its cell permeability. Key steps:
- Inject RBITC (1–5 mM in PBS) into target neuronal regions.
- Allow 24–72 hours for axonal transport.
- Fix tissues with paraformaldehyde and image using confocal microscopy with a 570 nm laser .
Advanced Research Questions
Q. How do mixed isomers of RBITC impact experimental reproducibility in protein labeling?
The mixed isomers (5- and 6- positions) exhibit variability in reactivity and fluorescence quantum yield. To minimize batch effects:
- Characterize isomer ratios using HPLC or mass spectrometry .
- Standardize conjugation protocols (pH, temperature, molar ratios) across experiments .
- Include internal controls (e.g., pre-conjugated protein standards) to normalize fluorescence intensity .
Q. What mechanisms underlie RBITC’s tumor-selective fluorescence activation?
RBITC’s fluorescence is quenched in neutral pH (healthy tissues) but activated in acidic tumor microenvironments. This property arises from protonation of its xanthene ring, which enhances quantum yield in low-pH conditions (e.g., pH < 6.5). Validate using:
- pH-calibrated fluorescence microscopy .
- Co-staining with pH-sensitive probes (e.g., FITC) in 3D tumor spheroid models .
Q. How can I design temperature-dependent fluorescence experiments using RBITC?
RBITC’s fluorescence intensity decreases linearly with temperature (quenching coefficient: ~1.5%/°C). Applications include:
- Mapping intracellular temperature gradients by correlating fluorescence intensity with calibrated thermal stages .
- Combining with thermosensitive nanoparticles for controlled drug release studies .
Q. How do solvent polarity and isomer ratios affect RBITC’s absorption spectrum?
Polar solvents (e.g., ethanol vs. DMSO) shift RBITC’s absorption maxima due to solvatochromism. For consistent results:
- Characterize solvent effects using UV-Vis spectroscopy .
- Use ratiometric measurements with a reference dye (e.g., FITC) in dual-labeling experiments .
Q. How can I resolve contradictory fluorescence data in RBITC-based multiplex assays?
Contradictions may arise from spectral overlap (e.g., with Texas Red) or pH-dependent quenching. Mitigation strategies:
- Use spectral unmixing software to deconvolve overlapping signals .
- Perform pH calibration in situ using buffer systems (e.g., HEPES vs. carbonate) .
Q. What advanced methods quantify RBITC conjugation efficiency in complex biological matrices?
- Chromatography : SEC-HPLC to separate conjugated vs. free RBITC .
- Mass spectrometry : Detect mass shifts in labeled proteins (e.g., +536 Da for RBITC) .
- Fluorescence correlation spectroscopy (FCS) : Measure labeled protein mobility in live cells .
Q. How can RBITC be integrated into pH-responsive drug delivery systems?
RBITC is co-condensed with FITC in mesoporous silica nanoparticles for ratiometric pH sensing:
Comparison with Similar Compounds
Fluorescein Isothiocyanate (FITC)
Property | RBITC | FITC |
---|---|---|
Excitation/Emission | 560 nm/580 nm | 495 nm/520 nm |
Quantum Yield (ΦF) | ~0.7 (estimated) | ~0.93 (in aqueous buffer) |
Reactivity | Amine-reactive (isothiocyanate) | Amine-reactive (isothiocyanate) |
Photostability | Moderate; slower fading | Low; rapid fading under UV |
Applications | Red channel imaging, protein tracking | Green channel imaging, immunofluorescence |
Key Advantages | Red emission minimizes autofluorescence | High brightness in green spectrum |
FITC, a green-emitting dye, suffers from rapid photobleaching under UV light compared to RBITC’s superior red-channel stability . However, FITC’s higher quantum yield enhances sensitivity in low-light conditions .
Tetramethylrhodamine Isothiocyanate (TRITC)
TRITC’s narrower emission spectrum makes it ideal for multiplexing, but its lower quantum yield reduces brightness compared to RBITC .
Texas Red (Sulforhodamine 101 Sulfonyl Chloride)
Property | RBITC | Texas Red |
---|---|---|
Excitation/Emission | 560 nm/580 nm | 595 nm/615 nm |
Reactivity | Amine-reactive | Sulfonyl chloride (reacts with thiols, amines) |
Photostability | Moderate | High |
Applications | Protein labeling, drug delivery | Far-red imaging, membrane studies |
Key Advantages | Cost-effective | Superior photostability and far-red emission |
Texas Red’s sulfonyl chloride group allows conjugation to multiple residues, but RBITC’s isothiocyanate offers simpler amine-specific labeling .
Alexa Fluor 594
Property | RBITC | Alexa Fluor 594 |
---|---|---|
Excitation/Emission | 560 nm/580 nm | 590 nm/617 nm |
Quantum Yield (ΦF) | ~0.7 | ~0.9 |
Photostability | Moderate | Extremely high |
Applications | General-purpose labeling | Super-resolution microscopy, live-cell imaging |
Key Differences | Lower cost, mixed isomers | Engineered for enhanced brightness and stability |
Alexa Fluor 594 outperforms RBITC in photostability and brightness due to proprietary structural modifications .
Lissamine Rhodamine B (RB 200)
Property | RBITC | RB 200 |
---|---|---|
Reactivity | Amine-reactive (isothiocyanate) | Sulfonyl chloride |
Emission | 580 nm | 575 nm |
Contrast in Staining | Moderate | Superior (post-chromatography) |
Applications | Protein conjugates | Dual-labeling with FITC |
RB 200’s sulfonyl chloride enables efficient post-chromatography purification, improving staining contrast compared to RBITC .
Critical Research Findings
Properties
IUPAC Name |
[9-(2-carboxy-4-isothiocyanatophenyl)-6-(diethylamino)xanthen-3-ylidene]-diethylazanium;[9-(2-carboxy-5-isothiocyanatophenyl)-6-(diethylamino)xanthen-3-ylidene]-diethylazanium;dichloride | |
---|---|---|
Source | PubChem | |
URL | https://pubchem.ncbi.nlm.nih.gov | |
Description | Data deposited in or computed by PubChem | |
InChI |
InChI=1S/2C29H29N3O3S.2ClH/c1-5-31(6-2)20-10-13-23-26(16-20)35-27-17-21(32(7-3)8-4)11-14-24(27)28(23)25-15-19(30-18-36)9-12-22(25)29(33)34;1-5-31(6-2)20-10-13-23-26(16-20)35-27-17-21(32(7-3)8-4)11-14-24(27)28(23)22-12-9-19(30-18-36)15-25(22)29(33)34;;/h2*9-17H,5-8H2,1-4H3;2*1H | |
Source | PubChem | |
URL | https://pubchem.ncbi.nlm.nih.gov | |
Description | Data deposited in or computed by PubChem | |
InChI Key |
ZCHFWDRVFPQJSN-UHFFFAOYSA-N | |
Source | PubChem | |
URL | https://pubchem.ncbi.nlm.nih.gov | |
Description | Data deposited in or computed by PubChem | |
Canonical SMILES |
CCN(CC)C1=CC2=C(C=C1)C(=C3C=CC(=[N+](CC)CC)C=C3O2)C4=C(C=C(C=C4)N=C=S)C(=O)O.CCN(CC)C1=CC2=C(C=C1)C(=C3C=CC(=[N+](CC)CC)C=C3O2)C4=C(C=CC(=C4)N=C=S)C(=O)O.[Cl-].[Cl-] | |
Source | PubChem | |
URL | https://pubchem.ncbi.nlm.nih.gov | |
Description | Data deposited in or computed by PubChem | |
Molecular Formula |
C58H60Cl2N6O6S2 | |
Source | PubChem | |
URL | https://pubchem.ncbi.nlm.nih.gov | |
Description | Data deposited in or computed by PubChem | |
Molecular Weight |
1072.2 g/mol | |
Source | PubChem | |
URL | https://pubchem.ncbi.nlm.nih.gov | |
Description | Data deposited in or computed by PubChem | |
Disclaimer and Information on In-Vitro Research Products
Please be aware that all articles and product information presented on BenchChem are intended solely for informational purposes. The products available for purchase on BenchChem are specifically designed for in-vitro studies, which are conducted outside of living organisms. In-vitro studies, derived from the Latin term "in glass," involve experiments performed in controlled laboratory settings using cells or tissues. It is important to note that these products are not categorized as medicines or drugs, and they have not received approval from the FDA for the prevention, treatment, or cure of any medical condition, ailment, or disease. We must emphasize that any form of bodily introduction of these products into humans or animals is strictly prohibited by law. It is essential to adhere to these guidelines to ensure compliance with legal and ethical standards in research and experimentation.