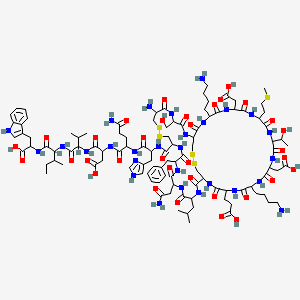
sarafotoxin S6a1
- Click on QUICK INQUIRY to receive a quote from our team of experts.
- With the quality product at a COMPETITIVE price, you can focus more on your research.
Overview
Description
Sarafotoxin S6a is a potent vasoconstrictor peptide found in the venom of the snake Atractaspis engaddensis . It belongs to the sarafotoxin family, which shares structural and functional similarities with endothelins, a group of peptides known for their strong vasoconstrictive properties . Sarafotoxin S6a is composed of twenty-one amino acid residues that fold into a defined tertiary structure stabilized by disulfide bonds .
Mechanism of Action
Target of Action
Sarafotoxin S6a is a sarafotoxin analogue that primarily targets the endothelin receptors , specifically the Endothelin Receptor Type A (ET A) and Endothelin Receptor Type B (ET B) . These receptors are G-protein-coupled receptors that play a crucial role in vasoconstriction, a process that narrows the blood vessels.
Mode of Action
Sarafotoxin S6a acts as an agonist to the endothelin receptors . It has an ET A/ET B selectivity profile similar to that of Endothelin-3 . This means that Sarafotoxin S6a can bind to these receptors and activate them, leading to a series of biochemical reactions that result in vasoconstriction .
Biochemical Pathways
Upon activation of the endothelin receptors by Sarafotoxin S6a, there is an induction of hydrolysis of phosphoinositides in the tissues . This suggests that Sarafotoxin S6a and endothelins use the phosphoinositide signal transduction pathway via specific receptors coupled to G protein . The activation of these pathways leads to various downstream effects, including vasoconstriction.
Result of Action
The primary result of Sarafotoxin S6a’s action is a rapid and marked vasoconstriction of the coronary vessels . This can lead to a decrease in blood flow and oxygen supply to the heart. In addition, it has been shown to cause a severe atrioventricular block and a slower but very strong positive inotropic effect in mice and rats .
Biochemical Analysis
Biochemical Properties
Sarafotoxin S6a is an endothelin receptor agonist . It has been shown to have a high affinity for endothelin receptors, specifically the ETA and ETB subtypes . The interaction between Sarafotoxin S6a and these receptors can lead to various biochemical reactions, such as the contraction of pig coronary artery .
Cellular Effects
Sarafotoxin S6a has been shown to have significant effects on various types of cells. For instance, it can decrease cardiac output by inducing left ventricular dysfunction . It can also increase airway pressures and lead to acute right ventricular dilatation .
Molecular Mechanism
The molecular mechanism of Sarafotoxin S6a involves its interaction with endothelin receptors. It binds with a high affinity to these receptors, leading to the induction of hydrolysis of phosphoinositides in atrial and brain membranes .
Temporal Effects in Laboratory Settings
In laboratory settings, Sarafotoxin S6a has been observed to have different effects over time. For example, it has been shown to have three independent effects in both mice and rats hearts: a rapid and marked vasoconstriction of the coronary vessels, a severe atrioventricular block, and a slower but very strong positive inotropic effect .
Dosage Effects in Animal Models
The effects of Sarafotoxin S6a can vary with different dosages in animal models. For instance, it has been shown to decrease cardiac output by inducing left ventricular dysfunction at one lethal dose (LD50), while at the same dosage, it can induce right ventricular dysfunction with increased airway pressures .
Preparation Methods
Synthetic Routes and Reaction Conditions: Sarafotoxin S6a can be synthesized using solid-phase peptide synthesis (SPPS), a method commonly employed for the production of peptides. The synthesis involves the stepwise addition of amino acids to a growing peptide chain anchored to a solid resin . The reaction conditions typically include the use of protecting groups to prevent unwanted side reactions and the formation of disulfide bonds to stabilize the peptide structure .
Industrial Production Methods: While there is limited information on the industrial production of Sarafotoxin S6a, it is likely that large-scale synthesis would follow similar principles as SPPS, with optimizations for yield and purity. The process would involve automated peptide synthesizers and rigorous purification steps, such as high-performance liquid chromatography (HPLC), to ensure the final product’s quality .
Chemical Reactions Analysis
Types of Reactions: Sarafotoxin S6a undergoes various chemical reactions, including:
Substitution: Amino acid residues within the peptide can be substituted to create analogs with altered biological activity.
Common Reagents and Conditions:
Oxidation: Air oxidation or the use of mild oxidizing agents like iodine can facilitate disulfide bond formation.
Reduction: Reducing agents such as DTT or β-mercaptoethanol are used under mild conditions to break disulfide bonds.
Substitution: Amino acid substitutions are typically performed during the SPPS process by incorporating different amino acids at specific positions.
Major Products Formed:
Scientific Research Applications
Sarafotoxin S6a has several scientific research applications, including:
Comparison with Similar Compounds
Sarafotoxin S6a is part of a family of peptides that includes other sarafotoxins and endothelins. Similar compounds include:
Sarafotoxin S6b: Another member of the sarafotoxin family with slight variations in amino acid sequence and biological activity.
Endothelin-1, Endothelin-2, Endothelin-3: Endogenous peptides with strong vasoconstrictive properties and structural similarities to sarafotoxins.
Uniqueness: Sarafotoxin S6a is unique due to its origin from snake venom and its specific amino acid sequence, which confers distinct biological properties . Its potent vasoconstrictive effects and high affinity for endothelin receptors make it a valuable tool in research and potential therapeutic applications .
Properties
CAS No. |
126738-34-9 |
---|---|
Molecular Formula |
C105H156N28O34S5 |
Molecular Weight |
2514.9 g/mol |
IUPAC Name |
3-[[5-amino-2-[[2-[[31-amino-7,22-bis(4-aminobutyl)-42-(2-amino-2-oxoethyl)-39-benzyl-4-(2-carboxyethyl)-10,19-bis(carboxymethyl)-13-(1-hydroxyethyl)-28-(hydroxymethyl)-45-(2-methylpropyl)-16-(2-methylsulfanylethyl)-3,6,9,12,15,18,21,24,27,30,38,41,44,47-tetradecaoxo-33,34,49,50-tetrathia-2,5,8,11,14,17,20,23,26,29,37,40,43,46-tetradecazabicyclo[23.22.4]henpentacontane-36-carbonyl]amino]-3-(1H-imidazol-4-yl)propanoyl]amino]-5-oxopentanoyl]amino]-4-[[1-[[1-[[1-carboxy-2-(1H-indol-3-yl)ethyl]amino]-3-methyl-1-oxopentan-2-yl]amino]-3-methyl-1-oxobutan-2-yl]amino]-4-oxobutanoic acid |
InChI |
InChI=1S/C105H156N28O34S5/c1-9-51(6)83(103(164)126-71(105(166)167)35-54-41-112-58-22-14-13-21-56(54)58)132-102(163)82(50(4)5)131-97(158)70(40-81(144)145)124-88(149)61(25-27-76(109)136)117-93(154)66(36-55-42-111-48-113-55)121-101(162)75-45-170-169-44-57(108)85(146)127-72(43-134)98(159)130-73-46-171-172-47-74(100(161)119-64(33-49(2)3)91(152)122-67(37-77(110)137)94(155)120-65(92(153)129-75)34-53-19-11-10-12-20-53)128-89(150)62(26-28-78(138)139)116-86(147)59(23-15-17-30-106)114-96(157)69(39-80(142)143)125-104(165)84(52(7)135)133-90(151)63(29-32-168-8)118-95(156)68(38-79(140)141)123-87(148)60(115-99(73)160)24-16-18-31-107/h10-14,19-22,41-42,48-52,57,59-75,82-84,112,134-135H,9,15-18,23-40,43-47,106-108H2,1-8H3,(H2,109,136)(H2,110,137)(H,111,113)(H,114,157)(H,115,160)(H,116,147)(H,117,154)(H,118,156)(H,119,161)(H,120,155)(H,121,162)(H,122,152)(H,123,148)(H,124,149)(H,125,165)(H,126,164)(H,127,146)(H,128,150)(H,129,153)(H,130,159)(H,131,158)(H,132,163)(H,133,151)(H,138,139)(H,140,141)(H,142,143)(H,144,145)(H,166,167) |
InChI Key |
BOQKWORWRAYXSI-UHFFFAOYSA-N |
SMILES |
CCC(C)C(C(=O)NC(CC1=CNC2=CC=CC=C21)C(=O)O)NC(=O)C(C(C)C)NC(=O)C(CC(=O)O)NC(=O)C(CCC(=O)N)NC(=O)C(CC3=CNC=N3)NC(=O)C4CSSCC(C(=O)NC(C(=O)NC5CSSCC(C(=O)NC(C(=O)NC(C(=O)NC(C(=O)N4)CC6=CC=CC=C6)CC(=O)N)CC(C)C)NC(=O)C(NC(=O)C(NC(=O)C(NC(=O)C(NC(=O)C(NC(=O)C(NC(=O)C(NC5=O)CCCCN)CC(=O)O)CCSC)C(C)O)CC(=O)O)CCCCN)CCC(=O)O)CO)N |
Canonical SMILES |
CCC(C)C(C(=O)NC(CC1=CNC2=CC=CC=C21)C(=O)O)NC(=O)C(C(C)C)NC(=O)C(CC(=O)O)NC(=O)C(CCC(=O)N)NC(=O)C(CC3=CNC=N3)NC(=O)C4CSSCC(C(=O)NC(C(=O)NC5CSSCC(C(=O)NC(C(=O)NC(C(=O)NC(C(=O)N4)CC6=CC=CC=C6)CC(=O)N)CC(C)C)NC(=O)C(NC(=O)C(NC(=O)C(NC(=O)C(NC(=O)C(NC(=O)C(NC(=O)C(NC5=O)CCCCN)CC(=O)O)CCSC)C(C)O)CC(=O)O)CCCCN)CCC(=O)O)CO)N |
Synonyms |
2,2'-((1R,2Z,4S,5E,7S,8Z,10S,11Z,13S,14Z,16S,17Z,19S,20Z,22S,23Z,25R,26Z,28S,29Z,31R,36R,37Z,39S,40Z,42S,43Z,45S,46Z)-36-((1Z,3S,4Z,6S,7Z,9S,10Z,12S,13Z,15S,16Z,18S)-3-((1H-imidazol-5-yl)methyl)-15-((S)-sec-butyl)-18-carboxy-9-(carboxymethyl)-1,4,7, |
Origin of Product |
United States |
Q1: How does Sarafotoxin S6a induce a nociceptive response in mice?
A: Sarafotoxin S6a, similar to Endothelin-1 and Endothelin-2, induces a nociceptive response in mice primarily by activating Endothelin receptors []. While both ETA and ETB receptors are involved in endothelin-mediated nociception, Sarafotoxin S6a exhibits a higher affinity for the ETA receptor subtype []. The exact mechanism by which ETA receptor activation leads to nociception requires further investigation, but it likely involves neuronal signaling pathways within the peripheral and/or central nervous system.
Q2: What structural features of Endothelin-1 are essential for its nociceptive effects, and how does this relate to Sarafotoxin S6a?
A: Research indicates that the C-terminal hexapeptide of Endothelin-1 alone is insufficient to induce a nociceptive response []. This suggests that other structural elements within the full Endothelin-1 molecule are crucial for receptor binding and activation leading to nociception. Interestingly, while Sarafotoxin S6a shares some structural similarities with Endothelins, it lacks the C-terminal tail present in Endothelin-1. This highlights that the structural requirements for activating Endothelin receptors to induce nociception might differ between Sarafotoxin S6a and Endothelin-1. Further research is needed to elucidate the precise binding sites and conformational changes induced by Sarafotoxin S6a on ETA receptors to elicit its nociceptive effects.
Disclaimer and Information on In-Vitro Research Products
Please be aware that all articles and product information presented on BenchChem are intended solely for informational purposes. The products available for purchase on BenchChem are specifically designed for in-vitro studies, which are conducted outside of living organisms. In-vitro studies, derived from the Latin term "in glass," involve experiments performed in controlled laboratory settings using cells or tissues. It is important to note that these products are not categorized as medicines or drugs, and they have not received approval from the FDA for the prevention, treatment, or cure of any medical condition, ailment, or disease. We must emphasize that any form of bodily introduction of these products into humans or animals is strictly prohibited by law. It is essential to adhere to these guidelines to ensure compliance with legal and ethical standards in research and experimentation.