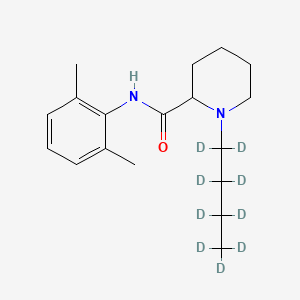
Bupivacaine-d9
Overview
Description
Bupivacaine-d9 is a deuterium-labeled analog of bupivacaine, a widely used local anesthetic. The deuterium labeling involves the replacement of hydrogen atoms with deuterium, a stable isotope of hydrogen. This modification is primarily used in scientific research to study the pharmacokinetics and metabolism of bupivacaine, as well as to serve as an internal standard in mass spectrometry.
Mechanism of Action
Target of Action
Bupivacaine-d9, like its parent compound Bupivacaine, primarily targets sodium channels on nerve cell membranes . These channels play a crucial role in the initiation and conduction of nerve impulses. By interacting with these channels, this compound can influence the transmission of signals in the nervous system .
Mode of Action
This compound acts by binding to sodium channels and inhibiting the influx of sodium ions . This action decreases the neuronal membrane’s permeability to sodium ions, resulting in inhibition of depolarization. Consequently, this blockade prevents the propagation of nerve impulses, leading to a loss of sensation in the targeted area .
Biochemical Pathways
This compound affects several biochemical pathways. For instance, it has been found to modulate apoptosis and ferroptosis in bladder cancer via the phosphatidylinositol 3-kinase (PI3K)/AKT pathway . Additionally, it has been reported to regulate the BDNF-TrkB/proBDNF-p75NTR pathway, which is involved in neuronal maturation and oxidative stress .
Pharmacokinetics
This compound, like Bupivacaine, is extensively and rapidly metabolized in the liver, followed by excretion via urine . Less than 10% of the dose is excreted as the parent compound, Bupivacaine . The pharmacokinetic properties of this compound are expected to be similar to those of Bupivacaine.
Result of Action
The primary result of this compound action is the temporary numbness or loss of sensation in specific areas of the body . On a molecular level, it has been found to induce apoptosis and ferroptosis in bladder cancer cells by inhibiting the PI3K/Akt signaling pathway . In non-small cell lung cancer, this compound has been reported to inhibit progression by inducing autophagy through the Akt/mTOR signaling pathway .
Action Environment
Environmental factors can influence the action, efficacy, and stability of this compound. For instance, the presence of other drugs, the patient’s metabolic state, and the specific physiological environment can impact the drug’s effectiveness . .
Biochemical Analysis
Biochemical Properties
Bupivacaine-d9 plays a significant role in biochemical reactions, particularly in the inhibition of sodium channels. It interacts with various enzymes, proteins, and other biomolecules. One of the primary interactions is with voltage-gated sodium channels, where this compound binds to the intracellular portion of the channel, inhibiting sodium ion influx and thereby blocking nerve impulse transmission . Additionally, this compound has been shown to interact with NMDA receptors, inhibiting their activity and contributing to its analgesic effects .
Cellular Effects
This compound affects various types of cells and cellular processes. In neurons, it inhibits sodium channels, leading to a decrease in action potential propagation and nerve signal transmission . This inhibition affects cell signaling pathways, particularly those involved in pain perception. This compound also influences gene expression by modulating the activity of transcription factors involved in inflammatory responses . Furthermore, it impacts cellular metabolism by altering the activity of enzymes involved in energy production and utilization.
Molecular Mechanism
The molecular mechanism of this compound involves its binding to voltage-gated sodium channels, leading to the inhibition of sodium ion influx . This binding occurs at the intracellular portion of the channel, stabilizing the inactivated state and preventing the generation and propagation of action potentials. Additionally, this compound inhibits NMDA receptor-mediated synaptic transmission by decreasing the open probability of the receptor channels . This dual mechanism contributes to its potent analgesic effects.
Temporal Effects in Laboratory Settings
In laboratory settings, the effects of this compound change over time. The compound is relatively stable, but its efficacy can decrease due to degradation. Studies have shown that this compound maintains its stability for several hours under controlled conditions . Long-term effects on cellular function include potential cytotoxicity at high concentrations, which can lead to cell death and impaired cellular processes . In vitro and in vivo studies have demonstrated that this compound can cause changes in cellular morphology and function over extended periods.
Dosage Effects in Animal Models
The effects of this compound vary with different dosages in animal models. At low doses, it provides effective local anesthesia without significant adverse effects . At higher doses, this compound can cause systemic toxicity, including neurotoxicity and cardiotoxicity . Studies in animal models have shown that the threshold for toxic effects is dose-dependent, with higher doses leading to more severe adverse effects. It is crucial to determine the appropriate dosage to balance efficacy and safety.
Metabolic Pathways
This compound is metabolized primarily in the liver, where it undergoes enzymatic degradation . The main metabolic pathways involve the cytochrome P450 enzymes, which hydroxylate the compound, leading to the formation of various metabolites. These metabolites are further conjugated and excreted in the urine. This compound also affects metabolic flux by altering the activity of enzymes involved in energy production and utilization . This can lead to changes in metabolite levels and overall cellular metabolism.
Transport and Distribution
This compound is transported and distributed within cells and tissues through passive diffusion and active transport mechanisms . It interacts with various transporters and binding proteins, which facilitate its movement across cell membranes. Once inside the cells, this compound can accumulate in specific compartments, such as the cytoplasm and mitochondria . This distribution pattern affects its localization and activity within the cells.
Subcellular Localization
The subcellular localization of this compound is primarily in the cytoplasm and mitochondria . It can also be found in the endoplasmic reticulum and other organelles, depending on the cell type and experimental conditions. The localization of this compound affects its activity and function, as it can interact with various biomolecules within these compartments. Post-translational modifications, such as phosphorylation, can also influence its targeting and localization within the cells .
Preparation Methods
Synthetic Routes and Reaction Conditions
The synthesis of Bupivacaine-d9 typically involves the deuteration of bupivacaine. This process can be achieved through various methods, including catalytic exchange reactions where hydrogen atoms in bupivacaine are replaced with deuterium atoms. One common method involves the use of deuterium gas (D2) in the presence of a catalyst such as palladium on carbon (Pd/C) under high pressure and temperature conditions.
Industrial Production Methods
Industrial production of this compound follows similar principles but on a larger scale. The process involves the use of specialized reactors and stringent quality control measures to ensure the purity and consistency of the deuterated compound. The final product is typically purified using techniques such as crystallization or chromatography to achieve the desired level of deuterium incorporation.
Chemical Reactions Analysis
Types of Reactions
Bupivacaine-d9, like its non-deuterated counterpart, can undergo various chemical reactions, including:
Oxidation: this compound can be oxidized to form corresponding N-oxides.
Reduction: Reduction reactions can convert this compound to its corresponding amine derivatives.
Substitution: Nucleophilic substitution reactions can occur at the amide nitrogen or aromatic ring.
Common Reagents and Conditions
Oxidation: Common oxidizing agents include hydrogen peroxide (H2O2) and m-chloroperbenzoic acid (m-CPBA).
Reduction: Reducing agents such as lithium aluminum hydride (LiAlH4) or sodium borohydride (NaBH4) are typically used.
Substitution: Reagents like sodium hydride (NaH) or potassium tert-butoxide (KOtBu) can facilitate nucleophilic substitution reactions.
Major Products
The major products formed from these reactions depend on the specific conditions and reagents used. For example, oxidation of this compound can yield N-oxides, while reduction can produce amine derivatives.
Scientific Research Applications
Bupivacaine-d9 is extensively used in scientific research due to its unique properties. Some of its applications include:
Pharmacokinetic Studies: this compound is used to study the absorption, distribution, metabolism, and excretion (ADME) of bupivacaine in biological systems.
Mass Spectrometry: It serves as an internal standard in gas chromatography-mass spectrometry (GC-MS) and liquid chromatography-mass spectrometry (LC-MS) for the quantification of bupivacaine.
Pain Research: this compound is used in studies investigating the mechanisms of pain and the efficacy of local anesthetics.
Neuroscience: It is employed in research on sodium channel blockers and their effects on neuronal signaling.
Comparison with Similar Compounds
Bupivacaine-d9 can be compared with other deuterated and non-deuterated local anesthetics, such as:
Lidocaine-d10: Another deuterium-labeled local anesthetic used for similar research purposes.
Ropivacaine: A non-deuterated local anesthetic with a similar mechanism of action but different pharmacokinetic properties.
Mepivacaine: Another local anesthetic with a shorter duration of action compared to bupivacaine.
This compound is unique due to its deuterium labeling, which provides advantages in pharmacokinetic studies and mass spectrometry applications, offering more precise and accurate measurements.
If you have any further questions or need additional details, feel free to ask!
Properties
IUPAC Name |
N-(2,6-dimethylphenyl)-1-(1,1,2,2,3,3,4,4,4-nonadeuteriobutyl)piperidine-2-carboxamide | |
---|---|---|
Source | PubChem | |
URL | https://pubchem.ncbi.nlm.nih.gov | |
Description | Data deposited in or computed by PubChem | |
InChI |
InChI=1S/C18H28N2O/c1-4-5-12-20-13-7-6-11-16(20)18(21)19-17-14(2)9-8-10-15(17)3/h8-10,16H,4-7,11-13H2,1-3H3,(H,19,21)/i1D3,4D2,5D2,12D2 | |
Source | PubChem | |
URL | https://pubchem.ncbi.nlm.nih.gov | |
Description | Data deposited in or computed by PubChem | |
InChI Key |
LEBVLXFERQHONN-JOJYFGIVSA-N | |
Source | PubChem | |
URL | https://pubchem.ncbi.nlm.nih.gov | |
Description | Data deposited in or computed by PubChem | |
Canonical SMILES |
CCCCN1CCCCC1C(=O)NC2=C(C=CC=C2C)C | |
Source | PubChem | |
URL | https://pubchem.ncbi.nlm.nih.gov | |
Description | Data deposited in or computed by PubChem | |
Isomeric SMILES |
[2H]C([2H])([2H])C([2H])([2H])C([2H])([2H])C([2H])([2H])N1CCCCC1C(=O)NC2=C(C=CC=C2C)C | |
Source | PubChem | |
URL | https://pubchem.ncbi.nlm.nih.gov | |
Description | Data deposited in or computed by PubChem | |
Molecular Formula |
C18H28N2O | |
Source | PubChem | |
URL | https://pubchem.ncbi.nlm.nih.gov | |
Description | Data deposited in or computed by PubChem | |
Molecular Weight |
297.5 g/mol | |
Source | PubChem | |
URL | https://pubchem.ncbi.nlm.nih.gov | |
Description | Data deposited in or computed by PubChem | |
Q1: Why is Bupivacaine-d9 chosen as an internal standard for Bupivacaine in this study?
A1: this compound is a deuterated form of Bupivacaine, meaning it possesses the same chemical structure with the exception of nine hydrogen atoms substituted by deuterium atoms. This isotopic substitution results in similar chemical behavior to Bupivacaine during sample preparation and analysis, but with a distinguishable mass difference detectable by mass spectrometry [].
Q2: How does the use of this compound contribute to the validation of the UPLC-MS/MS method?
A2: In the presented research [], the incorporation of this compound is essential for method validation, specifically contributing to:
Disclaimer and Information on In-Vitro Research Products
Please be aware that all articles and product information presented on BenchChem are intended solely for informational purposes. The products available for purchase on BenchChem are specifically designed for in-vitro studies, which are conducted outside of living organisms. In-vitro studies, derived from the Latin term "in glass," involve experiments performed in controlled laboratory settings using cells or tissues. It is important to note that these products are not categorized as medicines or drugs, and they have not received approval from the FDA for the prevention, treatment, or cure of any medical condition, ailment, or disease. We must emphasize that any form of bodily introduction of these products into humans or animals is strictly prohibited by law. It is essential to adhere to these guidelines to ensure compliance with legal and ethical standards in research and experimentation.