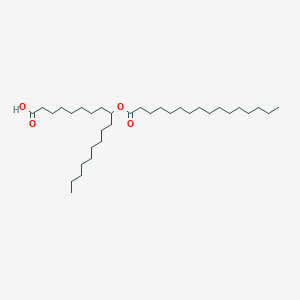
9-Pahsa
Overview
Description
9-Palmitic Acid Hydroxy Stearic Acid (9-PAHSA) is a member of the fatty acid esters of hydroxy fatty acids (FAHFAs) family. These compounds are endogenous bioactive lipids found in mammals, including humans. This compound has garnered significant attention due to its potential therapeutic benefits, particularly in metabolic and inflammatory diseases. It has been shown to improve glucose metabolism, reduce inflammation, and enhance insulin sensitivity .
Mechanism of Action
Target of Action
9-Pahsa, a member of the fatty acid esters of hydroxy fatty acids (FAHFA) family, is an endogenous mammalian bioactive lipid . It primarily targets chemokine G protein-coupled receptors (GPCRs), specifically CCR6, CCR7, CXCR4, and CXCR5 . These receptors play a crucial role in immune function and glucose metabolism .
Mode of Action
this compound interacts with its targets by displaying antagonist activity, which means it inhibits the action of these chemokine receptors . This interaction results in a modulation of immune function and glucose metabolism . It has also been found to stimulate insulin secretion and enhance glucose transport through the activation of GPR-120 .
Biochemical Pathways
The primary biochemical pathways affected by this compound involve glucose metabolism and immune function . It has been shown to decrease endogenous glucose production (EGP) through the inhibition of the cAMP/PKA signaling pathway, leading to decreased G6pase activity . Additionally, it has been found to down-regulate Akt/mTOR and activate the PI3KIII/BECN1 complex in diabetic myocardium .
Pharmacokinetics
It is known that this compound is present in circulation at nanomolar concentrations in mice and humans .
Result of Action
The action of this compound leads to several molecular and cellular effects. It has been reported to reduce blood glucose levels and attenuate inflammation . In addition, it has been shown to ameliorate carotid vascular calcification, promote autophagic flux, and reduce myocardial hypertrophy in db/db mice . Furthermore, it has been found to have a weak anti-inflammatory potential, as treatment reduced the LPS-induced secretion of certain chemokines .
Action Environment
The action, efficacy, and stability of this compound can be influenced by various environmental factors. For instance, diet can significantly impact its effects. In a study, long-term intake of this compound was found to modulate basal metabolism and improve insulin sensitivity in mice fed with a high fat-high sugar (HFHS) diet . No marked effect was observed on liver metabolism of obese diabetic mice . This suggests that the metabolic state of the individual can influence the action of this compound.
Biochemical Analysis
Biochemical Properties
9-Pahsa has been shown to interact with various enzymes, proteins, and other biomolecules. For instance, it has been found to inhibit lipolysis directly in white adipose tissue explants . It also interacts with Gα i protein–coupled receptors in a cAMP-dependent pathway .
Cellular Effects
This compound has been found to have significant effects on various types of cells and cellular processes. It has been shown to increase the viability of steatotic primary hepatocytes and HepG2 cells . It also prevents mitochondrial dysfunction and enhances insulin sensitivity .
Molecular Mechanism
This compound exerts its effects at the molecular level through various mechanisms. It inhibits basal and glucagon-stimulated endogenous glucose production directly in isolated hepatocytes through a cAMP-dependent pathway involving Gα i protein–coupled receptors .
Temporal Effects in Laboratory Settings
In laboratory settings, the effects of this compound have been observed to change over time. For instance, long-term intake of this compound has been shown to modulate basal metabolism favorably and enhance insulin sensitivity in mice .
Dosage Effects in Animal Models
The effects of this compound have been found to vary with different dosages in animal models. For example, daily oral administration of this compound to mice delayed the onset of type 1 diabetes and markedly reduced its incidence .
Metabolic Pathways
This compound is involved in various metabolic pathways. It has been found to modulate basal metabolism and improve insulin sensitivity . It also inhibits lipolysis directly in white adipose tissue explants .
Transport and Distribution
It is known that this compound can inhibit lipolysis directly in white adipose tissue explants, suggesting that it may interact with transporters or binding proteins in these cells .
Subcellular Localization
It is known that this compound can inhibit lipolysis directly in white adipose tissue explants, suggesting that it may be localized in these cells .
Preparation Methods
Synthetic Routes and Reaction Conditions
The synthesis of 9-PAHSA typically involves the esterification of palmitic acid with hydroxy stearic acid. The process can be summarized as follows:
Esterification Reaction: Palmitic acid is reacted with hydroxy stearic acid in the presence of a catalyst such as sulfuric acid or p-toluenesulfonic acid.
Purification: The reaction mixture is then purified using techniques such as column chromatography to isolate the desired product.
Industrial Production Methods
Industrial production of this compound follows similar principles but on a larger scale. The process involves:
Bulk Esterification: Large quantities of palmitic acid and hydroxy stearic acid are esterified in industrial reactors.
Purification and Quality Control: The product is purified using industrial-scale chromatography and subjected to rigorous quality control to ensure purity and consistency.
Chemical Reactions Analysis
Types of Reactions
9-PAHSA undergoes various chemical reactions, including:
Oxidation: this compound can be oxidized to form hydroperoxides and other oxidation products.
Reduction: Reduction reactions can convert this compound to its corresponding alcohols.
Substitution: Substitution reactions can occur at the ester linkage, leading to the formation of different ester derivatives.
Common Reagents and Conditions
Oxidation: Common oxidizing agents include hydrogen peroxide and potassium permanganate.
Reduction: Reducing agents such as lithium aluminum hydride or sodium borohydride are used.
Substitution: Acid or base catalysts are often employed to facilitate substitution reactions.
Major Products
The major products formed from these reactions include various oxidized, reduced, and substituted derivatives of this compound .
Scientific Research Applications
9-PAHSA has a wide range of scientific research applications:
Chemistry: It is used as a model compound to study the behavior of FAHFAs and their derivatives.
Biology: Research has shown that this compound plays a role in regulating glucose metabolism and insulin sensitivity.
Medicine: this compound has potential therapeutic applications in treating metabolic disorders such as diabetes and nonalcoholic fatty liver disease.
Industry: It is used in the development of bioactive lipid-based formulations for various applications
Comparison with Similar Compounds
Similar Compounds
- 9-Hydroxy Stearic Acid (9-HSA)
- 9-Palmitic Acid Hydroxy Palmitic Acid (9-PAHPA)
- 9-Oleic Acid Hydroxy Stearic Acid (9-OAHSA)
Uniqueness of 9-PAHSA
Compared to other similar compounds, this compound is unique due to its specific combination of palmitic acid and hydroxy stearic acid, which imparts distinct biological activities. It has shown superior efficacy in improving glucose metabolism and reducing inflammation compared to its analogs .
Biological Activity
9-Pahsa (Palmitic-acid-9-hydroxy-stearic-acid) is a recently identified endogenous lipid that has garnered attention for its potential therapeutic effects, particularly in the context of metabolic disorders and cardiovascular diseases. This article provides an in-depth exploration of the biological activity of this compound, focusing on its mechanisms of action, effects on glucose metabolism, anti-inflammatory properties, and implications for diabetic cardiovascular complications.
Overview of this compound
This compound is synthesized in the body and is notably elevated in adipose tissue of transgenic mice overexpressing glucose transporter 4 (GLUT4) . Research indicates that levels of this compound correlate positively with insulin sensitivity and are significantly lower in insulin-resistant individuals . This lipid has been shown to exert various biological activities through multiple pathways, making it a candidate for therapeutic interventions in metabolic and cardiovascular diseases.
1. Glucose Metabolism
This compound has been demonstrated to improve glucose homeostasis. In studies involving db/db mice (a model for type 2 diabetes), administration of this compound resulted in:
- Lowered Blood Glucose Levels : After two weeks of treatment, significant reductions in blood glucose levels were observed compared to vehicle-treated controls .
- Improved Glucose Tolerance : The area under the glucose excursion curve was reduced, indicating enhanced glucose tolerance .
Table 1: Effects of this compound on Glucose Metabolism
Parameter | Control Group (Vehicle) | This compound Treatment (50 mg/kg) |
---|---|---|
Blood Glucose Levels (mg/dL) | Elevated | Significantly Lowered |
Glucose Tolerance | Poor | Improved |
Insulin Levels | Unchanged | Unchanged |
2. Autophagy Regulation
Autophagy plays a crucial role in cellular homeostasis and metabolism. Research indicates that this compound promotes autophagic flux, which is beneficial for cardiac health:
- Increased Autolysosomes : Electron microscopy revealed that treatment with this compound led to a significant increase in autolysosome formation in myocardial cells .
- Western Blot Analysis : Results showed downregulation of the Akt/mTOR pathway and activation of the PI3KIII/BECN1 complex, facilitating autophagy in diabetic myocardium .
3. Anti-inflammatory Effects
The anti-inflammatory properties of this compound have been well-documented:
- Reduction of Inflammatory Cytokines : In vitro studies demonstrated that this compound inhibited lipopolysaccharide (LPS)-induced cytokine production, including IL-12 and TNFα .
- Adipose Tissue Inflammation : Administration of this compound reduced inflammation markers in adipose tissue from high-fat diet-fed mice .
Case Studies
Several studies have highlighted the therapeutic potential of this compound:
- Study on Diabetic Cardiomyopathy : A study conducted on db/db mice showed that continuous administration of this compound for four weeks ameliorated myocardial hypertrophy and dysfunction. Ultrasound imaging confirmed improvements in carotid vascular calcification .
- Browning of White Adipose Tissue : Another investigation revealed that this compound activates GPR120, promoting the browning of white adipose tissue, which is associated with increased thermogenic activity and improved metabolic profiles .
Properties
IUPAC Name |
9-hexadecanoyloxyoctadecanoic acid | |
---|---|---|
Source | PubChem | |
URL | https://pubchem.ncbi.nlm.nih.gov | |
Description | Data deposited in or computed by PubChem | |
InChI |
InChI=1S/C34H66O4/c1-3-5-7-9-11-12-13-14-15-16-18-23-27-31-34(37)38-32(28-24-20-17-10-8-6-4-2)29-25-21-19-22-26-30-33(35)36/h32H,3-31H2,1-2H3,(H,35,36) | |
Source | PubChem | |
URL | https://pubchem.ncbi.nlm.nih.gov | |
Description | Data deposited in or computed by PubChem | |
InChI Key |
MHQWHZLXDBVXML-UHFFFAOYSA-N | |
Source | PubChem | |
URL | https://pubchem.ncbi.nlm.nih.gov | |
Description | Data deposited in or computed by PubChem | |
Canonical SMILES |
CCCCCCCCCCCCCCCC(=O)OC(CCCCCCCCC)CCCCCCCC(=O)O | |
Source | PubChem | |
URL | https://pubchem.ncbi.nlm.nih.gov | |
Description | Data deposited in or computed by PubChem | |
Molecular Formula |
C34H66O4 | |
Source | PubChem | |
URL | https://pubchem.ncbi.nlm.nih.gov | |
Description | Data deposited in or computed by PubChem | |
DSSTOX Substance ID |
DTXSID101274990 | |
Record name | 9-[(1-Oxohexadecyl)oxy]octadecanoic acid | |
Source | EPA DSSTox | |
URL | https://comptox.epa.gov/dashboard/DTXSID101274990 | |
Description | DSSTox provides a high quality public chemistry resource for supporting improved predictive toxicology. | |
Molecular Weight |
538.9 g/mol | |
Source | PubChem | |
URL | https://pubchem.ncbi.nlm.nih.gov | |
Description | Data deposited in or computed by PubChem | |
Physical Description |
Solid | |
Record name | FAHFA(16:0/9-O-18:0) | |
Source | Human Metabolome Database (HMDB) | |
URL | http://www.hmdb.ca/metabolites/HMDB0112106 | |
Description | The Human Metabolome Database (HMDB) is a freely available electronic database containing detailed information about small molecule metabolites found in the human body. | |
Explanation | HMDB is offered to the public as a freely available resource. Use and re-distribution of the data, in whole or in part, for commercial purposes requires explicit permission of the authors and explicit acknowledgment of the source material (HMDB) and the original publication (see the HMDB citing page). We ask that users who download significant portions of the database cite the HMDB paper in any resulting publications. | |
CAS No. |
1481636-31-0 | |
Record name | 9-[(1-Oxohexadecyl)oxy]octadecanoic acid | |
Source | CAS Common Chemistry | |
URL | https://commonchemistry.cas.org/detail?cas_rn=1481636-31-0 | |
Description | CAS Common Chemistry is an open community resource for accessing chemical information. Nearly 500,000 chemical substances from CAS REGISTRY cover areas of community interest, including common and frequently regulated chemicals, and those relevant to high school and undergraduate chemistry classes. This chemical information, curated by our expert scientists, is provided in alignment with our mission as a division of the American Chemical Society. | |
Explanation | The data from CAS Common Chemistry is provided under a CC-BY-NC 4.0 license, unless otherwise stated. | |
Record name | 9-[(1-Oxohexadecyl)oxy]octadecanoic acid | |
Source | EPA DSSTox | |
URL | https://comptox.epa.gov/dashboard/DTXSID101274990 | |
Description | DSSTox provides a high quality public chemistry resource for supporting improved predictive toxicology. | |
Record name | FAHFA(16:0/9-O-18:0) | |
Source | Human Metabolome Database (HMDB) | |
URL | http://www.hmdb.ca/metabolites/HMDB0112106 | |
Description | The Human Metabolome Database (HMDB) is a freely available electronic database containing detailed information about small molecule metabolites found in the human body. | |
Explanation | HMDB is offered to the public as a freely available resource. Use and re-distribution of the data, in whole or in part, for commercial purposes requires explicit permission of the authors and explicit acknowledgment of the source material (HMDB) and the original publication (see the HMDB citing page). We ask that users who download significant portions of the database cite the HMDB paper in any resulting publications. | |
Q1: What is 9-PAHSA and what makes it significant?
A1: Palmitic acid ester of 9-hydroxystearic acid (this compound) is a member of a recently discovered class of endogenous lipids called Fatty Acid Esters of Hydroxy Fatty Acids (FAHFAs). This compound and other FAHFAs have shown promising anti-inflammatory and anti-diabetic effects in preclinical studies. [, , , , , ] These potential therapeutic benefits make this compound a subject of significant research interest.
Q2: How does this compound exert its effects on glucose metabolism?
A2: Research suggests that this compound improves systemic and hepatic insulin sensitivity, particularly in cases of insulin resistance. [] It achieves this by augmenting insulin action on endogenous glucose production (EGP), both directly and indirectly, involving communication between the liver and adipose tissue. [] One study identified GPR43 as a potential receptor mediating the direct effects of this compound on EGP reduction. []
Q3: Does this compound directly activate any receptors?
A3: Yes, this compound has been shown to activate GPR43 in a calcium flux assay. [] This activation appears to contribute to its ability to reduce hepatic glucose production. [] Additionally, this compound may exert some of its anti-inflammatory effects through interaction with a subset of chemokine receptors, such as CCR6, CCR7, CXCR4, and CXCR5, acting as an antagonist. []
Q4: What are the effects of this compound on adipose tissue?
A4: this compound appears to influence adipose tissue by promoting the "browning" of white fat. [] This process, known as browning, involves the conversion of white adipocytes (fat cells) into beige adipocytes, which exhibit increased energy expenditure and thermogenic capacity. Research also suggests that this compound stimulates triacylglycerol (TAG) / fatty acid (FA) cycling in white adipose tissue (WAT) by impacting both lipogenesis (fat synthesis) and lipolysis (fat breakdown). [] This impact on lipid metabolism contributes to the potential metabolic benefits associated with this compound.
Q5: Does the stereochemistry of this compound matter for its biological activity?
A5: Yes, research indicates that the R-enantiomer (R-9-PAHSA) is the predominant form found in adipose tissue and the one that accumulates in transgenic mice with elevated FAHFA levels. [] Studies on its biosynthesis and degradation suggest that the pathways involved are stereospecific, favoring the production of R-9-PAHSA. [] This finding highlights the importance of considering stereochemistry when studying the activity and metabolism of this compound.
Q6: How is this compound biosynthesized?
A6: While the exact enzymes responsible for PAHSA biosynthesis remain unknown, research suggests that its production in white adipose tissue is linked to de novo lipogenesis, a process regulated by carbohydrate-responsive element-binding protein (ChREBP). [] Interestingly, the nuclear factor erythroid 2-related factor 2 (Nrf2)-mediated antioxidant defense system, including proteins like peroxiredoxin 6 (Prdx6), appears to play a role in this biosynthesis. []
Q7: What is the role of the Nrf2 pathway in this compound biosynthesis?
A7: Studies have shown a link between the Nrf2-mediated antioxidant defense system and this compound biosynthesis. [] Transgenic overexpression of Nrf2 and deletion of Prdx6, a component of this system, both led to reduced levels of this compound in WAT. [] Conversely, inducing oxidative stress increased PAHSA levels. [] These findings suggest a potential connection between cellular redox balance, regulated by the Nrf2 pathway, and the biosynthesis of this compound.
Q8: Does this compound offer any protective effects against inflammatory conditions?
A8: Preclinical studies suggest that this compound demonstrates anti-inflammatory properties, particularly in the context of colitis. [] It appears to achieve this by regulating both innate and adaptive immune responses, protecting the gut mucosal barrier. [] Part of this protective effect may involve the activation of GPR120. [] Additionally, this compound has shown a weak anti-inflammatory potential in a human cellular model, reducing the secretion of certain pro-inflammatory chemokines like CXCL10, MIP-1 beta, and MCP. []
Q9: Has this compound shown any benefits in models of diabetic complications?
A9: Research suggests that this compound might offer benefits in the context of diabetic cardiovascular complications. [, ] One study showed that this compound administration improved cardiovascular function in diabetic mice by promoting autophagic flux (a cellular recycling process) and reducing myocardial hypertrophy (enlargement of the heart muscle). [, ] Another study indicated a potential neuroprotective effect of S-9-PAHSA in a mouse model of type 2 diabetes, attributed to its ability to suppress apoptosis and oxidative stress in neuronal cells. []
Q10: Are there any existing methods to measure this compound levels?
A10: Yes, researchers have developed a liquid chromatography-mass spectrometry (LC-MS) method for separating and quantifying both R- and S-enantiomers of this compound. [] This method allows for the accurate assessment of this compound levels in biological samples and has been crucial in understanding its distribution and metabolism.
Q11: What are the potential therapeutic applications of this compound?
A11: Given its anti-inflammatory and anti-diabetic properties observed in preclinical studies, this compound holds potential as a therapeutic agent for:
- Type 2 Diabetes: By improving insulin sensitivity and reducing hepatic glucose production. [, ]
- Inflammatory Bowel Disease: By mitigating inflammation and protecting the gut mucosal barrier. []
- Obesity and Metabolic Syndrome: By promoting the browning of white adipose tissue, potentially enhancing energy expenditure. []
- Cardiovascular complications in diabetes: By improving cardiac function and reducing myocardial hypertrophy. []
Disclaimer and Information on In-Vitro Research Products
Please be aware that all articles and product information presented on BenchChem are intended solely for informational purposes. The products available for purchase on BenchChem are specifically designed for in-vitro studies, which are conducted outside of living organisms. In-vitro studies, derived from the Latin term "in glass," involve experiments performed in controlled laboratory settings using cells or tissues. It is important to note that these products are not categorized as medicines or drugs, and they have not received approval from the FDA for the prevention, treatment, or cure of any medical condition, ailment, or disease. We must emphasize that any form of bodily introduction of these products into humans or animals is strictly prohibited by law. It is essential to adhere to these guidelines to ensure compliance with legal and ethical standards in research and experimentation.