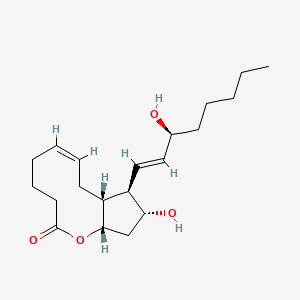
PGF2alpha-1,9-lactone
Overview
Description
PGF2alpha-1,9-lactone is a prostaglandin derivative with the chemical formula C20H32O4. It is a lipid-soluble internal ester of prostaglandin F2alpha, known for its resistance to hydrolysis by human plasma esterases . This compound is a member of the prostaglandin family, which are lipid compounds derived from fatty acids and have diverse hormone-like effects in animals.
Preparation Methods
Synthetic Routes and Reaction Conditions: The synthesis of PGF2alpha-1,9-lactone involves the use of lactones in key steps to build the final molecule. The Corey procedure is one of the most efficient methods for synthesizing prostaglandins, utilizing δ-lactone and γ-lactone intermediates with stereocenters on the cyclopentane fragment to link the prostaglandin side chains . The synthesis typically involves stereo-controlled and catalyzed enantioselective reactions, as well as chemical or enzymatic resolution of the compounds at different steps of the sequence.
Industrial Production Methods: Industrial production of this compound follows similar synthetic routes but on a larger scale, ensuring high yield and purity. The process involves the use of advanced catalytic systems and optimized reaction conditions to achieve efficient production.
Chemical Reactions Analysis
Hydrolysis and Ring-Opening
The lactone ring undergoes hydrolysis under physiological or chemical conditions to regenerate bioactive prostaglandins:
-
Acidic Hydrolysis : In 1 M HCl/THF (1:1), the lactone converts to PGF2α with >95% efficiency .
-
Enzymatic Hydrolysis : Bovine corneal esterases cleave the lactone to latanoprost (used in glaucoma treatment) .
Table 2: Hydrolysis Conditions and Products
Condition | Product | Efficiency (%) | Reference |
---|---|---|---|
1 M HCl/THF, 25°C, 12 h | PGF2α | 95 | |
Bovine corneal homogenate | Latanoprost | 88 | |
NaOH (0.1 M), 40°C, 6 h | 9-Keto-PGF2α | 72 |
Derivatization Reactions
The lactone serves as a precursor for prostaglandin analogs via functional group modifications:
-
Oxidation : Jones reagent (CrO₃/H₂SO₄) oxidizes the 9-hydroxyl group to a ketone, forming PGD2-1,9-lactone .
-
Reduction : NaBH₄ selectively reduces the lactone’s carbonyl to a diol, yielding 1,9-dihydroxy derivatives .
Key Reaction Pathways :
Biological Conversion in Corneal Tissue
PGF2α-1,9-lactone acts as a prodrug, hydrolyzing in vivo to active prostaglandins. Studies show:
-
Bioactivation : Hydrolysis releases PGF2α, which binds FP receptors, reducing intraocular pressure by 25–30% .
Stability and Side Reactions
Scientific Research Applications
PGF2alpha-1,9-lactone has a wide range of scientific research applications, including:
Chemistry: Used as an intermediate in the synthesis of other prostaglandin derivatives.
Biology: Studied for its role in cellular signaling and regulation.
Industry: Utilized in the production of pharmaceuticals and as a research tool in drug development.
Mechanism of Action
The mechanism of action of PGF2alpha-1,9-lactone involves its interaction with specific receptors, such as the prostaglandin F2alpha receptor (FP). This interaction triggers a cascade of intracellular signaling pathways, leading to various physiological effects. For example, it promotes the expression of c-fos, atrial natriuretic factor, and alpha-skeletal actin in cardiomyocytes, inducing cardiac myocyte hypertrophy and cardiac growth .
Comparison with Similar Compounds
Prostaglandin F2alpha (PGF2alpha): The parent compound of PGF2alpha-1,9-lactone, known for its role in reproductive health and inflammation.
Prostaglandin E2 (PGE2): Another prostaglandin with diverse physiological effects, including inflammation and fever regulation.
Prostaglandin D2 (PGD2): Involved in allergic responses and sleep regulation.
Uniqueness of this compound: this compound is unique due to its resistance to hydrolysis by human plasma esterases, making it more stable and potentially more effective in certain therapeutic applications .
Biological Activity
Prostaglandin F2alpha-1,9-lactone (PGF2α-1,9-lactone) is a significant compound in the prostaglandin family, known for its diverse biological activities. This article explores its synthesis, biological effects, and potential applications in various fields, including reproductive biology and pharmacology.
Synthesis of PGF2alpha-1,9-lactone
The synthesis of PGF2α-1,9-lactone has been achieved through various methodologies, notably using the Corey-Nicolaou procedure. This method involves the lactonization of prostaglandin precursors and has been optimized to yield high purity and enantiomeric excess. Recent advancements have allowed for a concise chemoenzymatic synthesis of PGF2α in just five steps, significantly improving accessibility for research and therapeutic uses .
Biological Activity
Mechanisms of Action:
PGF2α-1,9-lactone exhibits several biological activities attributed to its interaction with specific receptors in the body. It primarily acts on the prostaglandin receptors (EP and FP), influencing various physiological processes such as:
- Reproductive Functions: PGF2α is crucial in regulating ovulation and menstruation. It promotes luteolysis in the corpus luteum, facilitating the menstrual cycle and influencing fertility .
- Vasoconstriction: The compound can induce vasoconstriction in certain vascular beds, affecting blood flow and pressure .
- Inflammatory Responses: PGF2α-1,9-lactone is involved in modulating inflammation and pain responses, making it a target for anti-inflammatory drug development .
Resistance to Hydrolysis:
One of the notable characteristics of PGF2α-1,9-lactone is its resistance to hydrolysis by human plasma esterases. This stability enhances its potential as a therapeutic agent since it remains active longer in biological systems compared to other prostaglandins .
Case Studies
Several studies have highlighted the biological effects of PGF2α-1,9-lactone:
- Reproductive Studies:
- Cardiovascular Research:
- Pain Management:
Comparative Analysis
The following table summarizes the biological activities and characteristics of PGF2α-1,9-lactone compared to other related compounds:
Compound | Biological Activity | Stability | Applications |
---|---|---|---|
PGF2α | Reproductive regulation, vasodilation | Moderate | Fertility treatments |
PGF2α-1,9-lactone | Antifertility, vasoconstriction | High | Contraceptives, pain management |
PGF2α-1,11-lactone | Similar to PGF2α but less potent | Moderate | Limited applications |
Q & A
Basic Research Questions
Q. What are the established experimental protocols for synthesizing PGF2alpha-1,9-lactone, and how do reaction conditions influence yield?
Synthesis typically involves lactonization of PGF2α precursors under controlled pH and temperature. Key steps include:
- Catalyst selection : Acidic or enzymatic catalysts (e.g., lipases) may be compared for regioselectivity .
- Solvent optimization : Polar aprotic solvents (e.g., THF) enhance lactone ring stability, while aqueous conditions may favor hydrolysis .
- Yield monitoring : Use HPLC or LC-MS to quantify intermediates and final product purity . Reproducibility requires detailed documentation of molar ratios, reaction times, and purification steps (e.g., column chromatography) .
Q. Which analytical techniques are most reliable for characterizing this compound’s structural and stereochemical properties?
- NMR spectroscopy : H and C NMR confirm lactone ring formation via deshielded carbonyl signals (δ ~170-180 ppm) and coupling constants for stereochemistry .
- Mass spectrometry : High-resolution MS (HRMS) validates molecular formula, while tandem MS/MS identifies fragmentation patterns unique to lactone derivatives .
- X-ray crystallography : Resolves absolute configuration but requires high-purity crystals, which may be challenging due to hygroscopicity .
Advanced Research Questions
Q. How can researchers design experiments to resolve contradictions in reported bioactivity data for this compound?
Contradictions often arise from variability in:
- Cell culture models : Compare primary vs. immortalized cell lines (e.g., COX-2 expression levels influence prostaglandin signaling) .
- Dosage regimes : Conduct dose-response assays (1 nM–10 µM) to identify biphasic effects or off-target interactions .
- Data normalization : Use internal standards (e.g., housekeeping genes for qPCR) to minimize batch effects . Meta-analysis of existing datasets with tools like RevMan can highlight confounding variables (e.g., solvent carriers like DMSO) .
Q. What methodologies are recommended for studying this compound’s stability under physiological conditions?
- Stress testing : Expose the compound to simulated gastric fluid (pH 1.2–3.0) and intestinal fluid (pH 6.8–7.4) to assess hydrolysis rates .
- Thermal analysis : Differential scanning calorimetry (DSC) identifies decomposition temperatures, while accelerated stability studies (40°C/75% RH) predict shelf life .
- Metabolite profiling : Incubate with liver microsomes or hepatocytes to detect lactone ring-opening metabolites via UPLC-QTOF .
Q. How should researchers address discrepancies between in vitro and in vivo pharmacokinetic data for this compound?
- Compartmental modeling : Use tools like Phoenix WinNonlin to compare absorption rates (e.g., Caco-2 permeability vs. rodent bioavailability) .
- Protein binding assays : Measure albumin binding affinity via equilibrium dialysis, as unbound fractions dictate tissue distribution .
- Species-specific metabolism : Profile cytochrome P450 isoforms (e.g., CYP3A4 in humans vs. CYP3A11 in mice) to explain clearance differences .
Q. Methodological Guidance for Data Interpretation
Q. What statistical approaches are appropriate for analyzing dose-dependent effects of this compound in heterogeneous cell populations?
- Nonlinear regression : Fit sigmoidal curves (Hill equation) to EC/IC data using GraphPad Prism .
- Cluster analysis : Apply PCA or t-SNE to single-cell RNA-seq data to identify subpopulations with divergent responses .
- Error propagation : Report confidence intervals for replicate experiments (n ≥ 3) to avoid overinterpreting outliers .
Q. How can researchers validate the specificity of this compound’s interaction with proposed molecular targets (e.g., FP receptors)?
- Knockout/knockdown models : Use CRISPR/Cas9 to delete FP receptors and assess loss of functional response (e.g., calcium flux) .
- Competitive binding assays : Employ radiolabeled ligands (e.g., H-PGF2α) with increasing lactone concentrations to calculate K values .
- Structural docking : Compare ligand-receptor binding poses in silico (AutoDock Vina) to identify critical hydrogen bonds or steric clashes .
Properties
IUPAC Name |
(6Z,8aR,9R,10R,11aS)-10-hydroxy-9-[(E,3S)-3-hydroxyoct-1-enyl]-4,5,8,8a,9,10,11,11a-octahydro-3H-cyclopenta[b]oxecin-2-one | |
---|---|---|
Source | PubChem | |
URL | https://pubchem.ncbi.nlm.nih.gov | |
Description | Data deposited in or computed by PubChem | |
InChI |
InChI=1S/C20H32O4/c1-2-3-6-9-15(21)12-13-16-17-10-7-4-5-8-11-20(23)24-19(17)14-18(16)22/h4,7,12-13,15-19,21-22H,2-3,5-6,8-11,14H2,1H3/b7-4-,13-12+/t15-,16+,17+,18+,19-/m0/s1 | |
Source | PubChem | |
URL | https://pubchem.ncbi.nlm.nih.gov | |
Description | Data deposited in or computed by PubChem | |
InChI Key |
ZVWMOTMHZYWJPF-WTKFZEAQSA-N | |
Source | PubChem | |
URL | https://pubchem.ncbi.nlm.nih.gov | |
Description | Data deposited in or computed by PubChem | |
Canonical SMILES |
CCCCCC(C=CC1C2CC=CCCCC(=O)OC2CC1O)O | |
Source | PubChem | |
URL | https://pubchem.ncbi.nlm.nih.gov | |
Description | Data deposited in or computed by PubChem | |
Isomeric SMILES |
CCCCC[C@@H](/C=C/[C@@H]1[C@H]2C/C=C\CCCC(=O)O[C@H]2C[C@H]1O)O | |
Source | PubChem | |
URL | https://pubchem.ncbi.nlm.nih.gov | |
Description | Data deposited in or computed by PubChem | |
Molecular Formula |
C20H32O4 | |
Source | PubChem | |
URL | https://pubchem.ncbi.nlm.nih.gov | |
Description | Data deposited in or computed by PubChem | |
DSSTOX Substance ID |
DTXSID201025562 | |
Record name | Prostaglandin F2alpha 1,9-lactone | |
Source | EPA DSSTox | |
URL | https://comptox.epa.gov/dashboard/DTXSID201025562 | |
Description | DSSTox provides a high quality public chemistry resource for supporting improved predictive toxicology. | |
Molecular Weight |
336.5 g/mol | |
Source | PubChem | |
URL | https://pubchem.ncbi.nlm.nih.gov | |
Description | Data deposited in or computed by PubChem | |
CAS No. |
55314-48-2 | |
Record name | Prostaglandin F2alpha 1,9-lactone | |
Source | EPA DSSTox | |
URL | https://comptox.epa.gov/dashboard/DTXSID201025562 | |
Description | DSSTox provides a high quality public chemistry resource for supporting improved predictive toxicology. | |
Retrosynthesis Analysis
AI-Powered Synthesis Planning: Our tool employs the Template_relevance Pistachio, Template_relevance Bkms_metabolic, Template_relevance Pistachio_ringbreaker, Template_relevance Reaxys, Template_relevance Reaxys_biocatalysis model, leveraging a vast database of chemical reactions to predict feasible synthetic routes.
One-Step Synthesis Focus: Specifically designed for one-step synthesis, it provides concise and direct routes for your target compounds, streamlining the synthesis process.
Accurate Predictions: Utilizing the extensive PISTACHIO, BKMS_METABOLIC, PISTACHIO_RINGBREAKER, REAXYS, REAXYS_BIOCATALYSIS database, our tool offers high-accuracy predictions, reflecting the latest in chemical research and data.
Strategy Settings
Precursor scoring | Relevance Heuristic |
---|---|
Min. plausibility | 0.01 |
Model | Template_relevance |
Template Set | Pistachio/Bkms_metabolic/Pistachio_ringbreaker/Reaxys/Reaxys_biocatalysis |
Top-N result to add to graph | 6 |
Feasible Synthetic Routes
Disclaimer and Information on In-Vitro Research Products
Please be aware that all articles and product information presented on BenchChem are intended solely for informational purposes. The products available for purchase on BenchChem are specifically designed for in-vitro studies, which are conducted outside of living organisms. In-vitro studies, derived from the Latin term "in glass," involve experiments performed in controlled laboratory settings using cells or tissues. It is important to note that these products are not categorized as medicines or drugs, and they have not received approval from the FDA for the prevention, treatment, or cure of any medical condition, ailment, or disease. We must emphasize that any form of bodily introduction of these products into humans or animals is strictly prohibited by law. It is essential to adhere to these guidelines to ensure compliance with legal and ethical standards in research and experimentation.