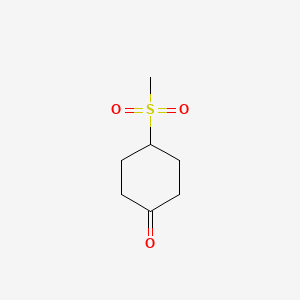
4-(Methylsulfonyl)cyclohexanone
Overview
Description
4-(Methylsulfonyl)cyclohexanone is an organic compound with the molecular formula C7H12O3S. It is characterized by a cyclohexanone ring substituted with a methylsulfonyl group at the fourth position.
Preparation Methods
Synthetic Routes and Reaction Conditions
The synthesis of 4-(Methylsulfonyl)cyclohexanone typically involves the introduction of a methylsulfonyl group to a cyclohexanone precursor. One common method is the reaction of cyclohexanone with methylsulfonyl chloride in the presence of a base such as triethylamine. The reaction is carried out under reflux conditions to ensure complete conversion .
Industrial Production Methods
In an industrial setting, the production of this compound may involve continuous flow processes to enhance efficiency and yield. The use of catalysts and optimized reaction conditions can further improve the scalability of the synthesis .
Chemical Reactions Analysis
Types of Reactions
4-(Methylsulfonyl)cyclohexanone undergoes various chemical reactions, including:
Oxidation: The compound can be oxidized to form sulfone derivatives.
Reduction: Reduction reactions can convert the ketone group to an alcohol.
Substitution: The methylsulfonyl group can be substituted with other functional groups under appropriate conditions.
Common Reagents and Conditions
Oxidation: Common oxidizing agents include potassium permanganate and hydrogen peroxide.
Reduction: Reducing agents such as sodium borohydride or lithium aluminum hydride are used.
Substitution: Nucleophilic substitution reactions can be carried out using reagents like sodium methoxide.
Major Products Formed
The major products formed from these reactions include sulfone derivatives, alcohols, and substituted cyclohexanones, depending on the reaction conditions and reagents used .
Scientific Research Applications
4-(Methylsulfonyl)cyclohexanone has several applications in scientific research:
Chemistry: It is used as an intermediate in the synthesis of various organic compounds.
Biology: The compound is studied for its potential biological activities and interactions with biomolecules.
Medicine: Research is ongoing to explore its potential therapeutic applications.
Industry: It is used in the production of specialty chemicals and materials.
Mechanism of Action
The mechanism of action of 4-(Methylsulfonyl)cyclohexanone involves its interaction with specific molecular targets. The methylsulfonyl group can participate in various chemical reactions, influencing the compound’s reactivity and interactions with other molecules. The ketone group can undergo nucleophilic addition reactions, further contributing to its chemical behavior .
Comparison with Similar Compounds
Similar Compounds
Cyclohexanone: A simple ketone with a cyclohexane ring.
4-(Methylthio)cyclohexanone: Similar structure but with a methylthio group instead of a methylsulfonyl group.
4-(Methanesulfonyl)cyclohexanone: Similar structure with a methanesulfonyl group.
Uniqueness
4-(Methylsulfonyl)cyclohexanone is unique due to the presence of the methylsulfonyl group, which imparts distinct chemical properties and reactivity compared to other similar compounds. This uniqueness makes it valuable for specific applications in research and industry .
Properties
IUPAC Name |
4-methylsulfonylcyclohexan-1-one | |
---|---|---|
Source | PubChem | |
URL | https://pubchem.ncbi.nlm.nih.gov | |
Description | Data deposited in or computed by PubChem | |
InChI |
InChI=1S/C7H12O3S/c1-11(9,10)7-4-2-6(8)3-5-7/h7H,2-5H2,1H3 | |
Source | PubChem | |
URL | https://pubchem.ncbi.nlm.nih.gov | |
Description | Data deposited in or computed by PubChem | |
InChI Key |
HZDUWRDWIUHEME-UHFFFAOYSA-N | |
Source | PubChem | |
URL | https://pubchem.ncbi.nlm.nih.gov | |
Description | Data deposited in or computed by PubChem | |
Canonical SMILES |
CS(=O)(=O)C1CCC(=O)CC1 | |
Source | PubChem | |
URL | https://pubchem.ncbi.nlm.nih.gov | |
Description | Data deposited in or computed by PubChem | |
Molecular Formula |
C7H12O3S | |
Source | PubChem | |
URL | https://pubchem.ncbi.nlm.nih.gov | |
Description | Data deposited in or computed by PubChem | |
DSSTOX Substance ID |
DTXSID301303438 | |
Record name | 4-(Methylsulfonyl)cyclohexanone | |
Source | EPA DSSTox | |
URL | https://comptox.epa.gov/dashboard/DTXSID301303438 | |
Description | DSSTox provides a high quality public chemistry resource for supporting improved predictive toxicology. | |
Molecular Weight |
176.24 g/mol | |
Source | PubChem | |
URL | https://pubchem.ncbi.nlm.nih.gov | |
Description | Data deposited in or computed by PubChem | |
CAS No. |
862129-72-4 | |
Record name | 4-(Methylsulfonyl)cyclohexanone | |
Source | CAS Common Chemistry | |
URL | https://commonchemistry.cas.org/detail?cas_rn=862129-72-4 | |
Description | CAS Common Chemistry is an open community resource for accessing chemical information. Nearly 500,000 chemical substances from CAS REGISTRY cover areas of community interest, including common and frequently regulated chemicals, and those relevant to high school and undergraduate chemistry classes. This chemical information, curated by our expert scientists, is provided in alignment with our mission as a division of the American Chemical Society. | |
Explanation | The data from CAS Common Chemistry is provided under a CC-BY-NC 4.0 license, unless otherwise stated. | |
Record name | 4-(Methylsulfonyl)cyclohexanone | |
Source | EPA DSSTox | |
URL | https://comptox.epa.gov/dashboard/DTXSID301303438 | |
Description | DSSTox provides a high quality public chemistry resource for supporting improved predictive toxicology. | |
Synthesis routes and methods
Procedure details
Disclaimer and Information on In-Vitro Research Products
Please be aware that all articles and product information presented on BenchChem are intended solely for informational purposes. The products available for purchase on BenchChem are specifically designed for in-vitro studies, which are conducted outside of living organisms. In-vitro studies, derived from the Latin term "in glass," involve experiments performed in controlled laboratory settings using cells or tissues. It is important to note that these products are not categorized as medicines or drugs, and they have not received approval from the FDA for the prevention, treatment, or cure of any medical condition, ailment, or disease. We must emphasize that any form of bodily introduction of these products into humans or animals is strictly prohibited by law. It is essential to adhere to these guidelines to ensure compliance with legal and ethical standards in research and experimentation.