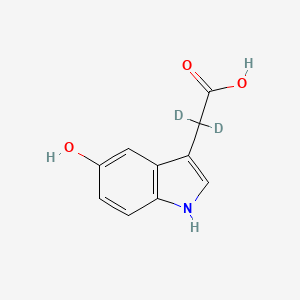
5-Hydroxyindole-3-acetic-2,2-d2 acid
Overview
Description
5-Hydroxyindole-3-acetic Acid-D2 is a labelled analogue of 5-Hydroxyindole-3-acetic Acid. 5-Hydroxyindole-3-acetic Acid is an impurity of Chlorpheniramine, a histamine H1 receptor antagonist that is used to treat allergy symptoms.
Mechanism of Action
Target of Action
5-Hydroxyindole-3-acetic Acid-D2, also known as 5-Hydroxyindole-3-acetic-2,2-d2 acid, is a deuterium-labeled derivative of 5-Hydroxyindole-3-acetic acid . This compound is a major metabolite of serotonin , a neurotransmitter that plays a crucial role in various biological activities, including mood regulation, gastrointestinal motility, and cardiovascular function . The primary targets of this compound are L-type calcium channels located on the colonic smooth muscle cells .
Mode of Action
The compound interacts with its targets, the L-type calcium channels, to stimulate intestinal motility . This interaction results in the acceleration of gut contractility . Moreover, the compound can stimulate a cell line model of intestinal enterochromaffin cells, resulting in a significant increase in serotonin production .
Biochemical Pathways
5-Hydroxyindole-3-acetic Acid-D2 is part of the serotonin pathway, where it serves as a catabolic end product . This pathway involves the metabolism of the dietary supplement and antidepressant 5-hydroxytryptophan (5-HTP) to 5-hydroxyindole (5-HI) via the tryptophanase (TnaA) enzyme .
Pharmacokinetics
The pharmacokinetics of 5-Hydroxyindole-3-acetic Acid-D2 is influenced by its deuterium labeling. Deuteration has gained attention for its potential to affect the pharmacokinetic and metabolic profiles of drugs . .
Result of Action
The action of 5-Hydroxyindole-3-acetic Acid-D2 leads to significant physiological effects. When administered orally in rats, it significantly accelerates the total gut transit time . This acceleration of gut contractility can potentially influence the overall gastrointestinal function.
Action Environment
The action of 5-Hydroxyindole-3-acetic Acid-D2 is influenced by various environmental factors. For instance, the production of 5-HI, a metabolite in the same pathway, is inhibited upon pH reduction in in vitro studies . Moreover, the compound’s action can be influenced by the gut microbiota, which plays a role in metabolizing 5-HTP to 5-HI .
Biochemical Analysis
Biochemical Properties
5-Hydroxyindole-3-acetic Acid-D2 plays a significant role in biochemical reactions. It is formed when serotonin is metabolized by monamine oxidase and aldehyde dehydrogenase in the liver . It interacts with various enzymes, proteins, and other biomolecules. For instance, it interacts with the enzyme tryptophanase (TnaA), which is involved in the conversion of the dietary supplement and antidepressant 5-hydroxytryptophan (5-HTP) to 5-hydroxyindole .
Cellular Effects
5-Hydroxyindole-3-acetic Acid-D2 has profound effects on various types of cells and cellular processes. It influences cell function by modulating cell signaling pathways, gene expression, and cellular metabolism . For instance, it has been found to accelerate gut contractility via activation of L-type calcium channels located on the colonic smooth muscle cells .
Molecular Mechanism
The molecular mechanism of action of 5-Hydroxyindole-3-acetic Acid-D2 involves its binding interactions with biomolecules, enzyme inhibition or activation, and changes in gene expression . It exerts its effects at the molecular level, for example, by accelerating gut contractility via activation of L-type calcium channels .
Temporal Effects in Laboratory Settings
In laboratory settings, the effects of 5-Hydroxyindole-3-acetic Acid-D2 change over time. It has been found that plasma 5-Hydroxyindole-3-acetic Acid-D2 levels were significantly elevated in severe Acute Respiratory Distress Syndrome (ARDS) cases with shock status and positively correlated with clinical severity .
Dosage Effects in Animal Models
The effects of 5-Hydroxyindole-3-acetic Acid-D2 vary with different dosages in animal models. For instance, when administered orally in rats, 5-Hydroxyindole-3-acetic Acid-D2 significantly accelerates the total gut transit time .
Metabolic Pathways
5-Hydroxyindole-3-acetic Acid-D2 is involved in the metabolism of serotonin, a process that involves several enzymes and cofactors . It is formed when serotonin is metabolized by monamine oxidase and aldehyde dehydrogenase in the liver .
Transport and Distribution
It is known that it is a metabolite of serotonin and is used to determine serotonin levels in the body .
Subcellular Localization
As a metabolite of serotonin, it is likely to be found wherever serotonin and its metabolites are localized within the cell .
Properties
IUPAC Name |
2,2-dideuterio-2-(5-hydroxy-1H-indol-3-yl)acetic acid | |
---|---|---|
Source | PubChem | |
URL | https://pubchem.ncbi.nlm.nih.gov | |
Description | Data deposited in or computed by PubChem | |
InChI |
InChI=1S/C10H9NO3/c12-7-1-2-9-8(4-7)6(5-11-9)3-10(13)14/h1-2,4-5,11-12H,3H2,(H,13,14)/i3D2 | |
Source | PubChem | |
URL | https://pubchem.ncbi.nlm.nih.gov | |
Description | Data deposited in or computed by PubChem | |
InChI Key |
DUUGKQCEGZLZNO-SMZGMGDZSA-N | |
Source | PubChem | |
URL | https://pubchem.ncbi.nlm.nih.gov | |
Description | Data deposited in or computed by PubChem | |
Canonical SMILES |
C1=CC2=C(C=C1O)C(=CN2)CC(=O)O | |
Source | PubChem | |
URL | https://pubchem.ncbi.nlm.nih.gov | |
Description | Data deposited in or computed by PubChem | |
Isomeric SMILES |
[2H]C([2H])(C1=CNC2=C1C=C(C=C2)O)C(=O)O | |
Source | PubChem | |
URL | https://pubchem.ncbi.nlm.nih.gov | |
Description | Data deposited in or computed by PubChem | |
Molecular Formula |
C10H9NO3 | |
Source | PubChem | |
URL | https://pubchem.ncbi.nlm.nih.gov | |
Description | Data deposited in or computed by PubChem | |
Molecular Weight |
193.20 g/mol | |
Source | PubChem | |
URL | https://pubchem.ncbi.nlm.nih.gov | |
Description | Data deposited in or computed by PubChem | |
Retrosynthesis Analysis
AI-Powered Synthesis Planning: Our tool employs the Template_relevance Pistachio, Template_relevance Bkms_metabolic, Template_relevance Pistachio_ringbreaker, Template_relevance Reaxys, Template_relevance Reaxys_biocatalysis model, leveraging a vast database of chemical reactions to predict feasible synthetic routes.
One-Step Synthesis Focus: Specifically designed for one-step synthesis, it provides concise and direct routes for your target compounds, streamlining the synthesis process.
Accurate Predictions: Utilizing the extensive PISTACHIO, BKMS_METABOLIC, PISTACHIO_RINGBREAKER, REAXYS, REAXYS_BIOCATALYSIS database, our tool offers high-accuracy predictions, reflecting the latest in chemical research and data.
Strategy Settings
Precursor scoring | Relevance Heuristic |
---|---|
Min. plausibility | 0.01 |
Model | Template_relevance |
Template Set | Pistachio/Bkms_metabolic/Pistachio_ringbreaker/Reaxys/Reaxys_biocatalysis |
Top-N result to add to graph | 6 |
Feasible Synthetic Routes
Q1: Why is 5-Hydroxyindole-3-acetic-2,2-d2 acid used in the analysis of 5-HIAA?
A1: this compound is a deuterated form of 5-HIAA, meaning it has nearly identical chemical properties but a slightly higher molecular weight due to the presence of two deuterium atoms. This makes it ideal for use as an internal standard in mass spectrometry analysis.
- Improved accuracy and precision: By adding a known amount of the internal standard to each sample, variations during sample preparation and analysis can be accounted for. This is because the internal standard will experience the same losses or variations as the analyte (5-HIAA), allowing for more accurate quantification. []
- Enhanced sensitivity: The use of a deuterated internal standard allows for selective detection and quantification of the target analyte even in complex matrices like urine. []
Q2: How does the use of this compound improve the linearity of the assay compared to the previous LC-EC method?
A2: While the research paper doesn't explicitly state the reason for improved linearity with the LC-MS/MS method using this compound compared to the previous LC-EC method, it's likely due to the inherent advantages of mass spectrometry coupled with the use of an internal standard.
- Wider dynamic range of detection: Mass spectrometry offers a broader linear range for detection compared to electrochemical detection methods. This means the assay can accurately quantify 5-HIAA over a wider range of concentrations. []
- Reduced matrix effects: Urine is a complex matrix, and components within it can interfere with the detection of analytes. Using an internal standard helps correct for these matrix effects, leading to a more accurate and linear response. []
Disclaimer and Information on In-Vitro Research Products
Please be aware that all articles and product information presented on BenchChem are intended solely for informational purposes. The products available for purchase on BenchChem are specifically designed for in-vitro studies, which are conducted outside of living organisms. In-vitro studies, derived from the Latin term "in glass," involve experiments performed in controlled laboratory settings using cells or tissues. It is important to note that these products are not categorized as medicines or drugs, and they have not received approval from the FDA for the prevention, treatment, or cure of any medical condition, ailment, or disease. We must emphasize that any form of bodily introduction of these products into humans or animals is strictly prohibited by law. It is essential to adhere to these guidelines to ensure compliance with legal and ethical standards in research and experimentation.