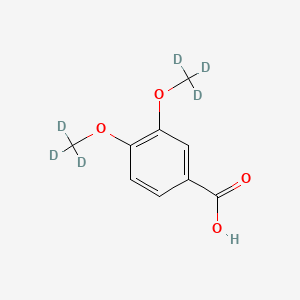
Veratric Acid-d6
Overview
Description
Veratric Acid-d6 (3,4-dimethoxybenzoic acid-d6) is a deuterium-labeled analog of veratric acid, a naturally occurring phenolic acid. Its molecular formula is C₉H₄D₆O₄, with six deuterium atoms replacing hydrogen atoms at specific positions. This isotopic modification increases its molecular mass without altering its chemical reactivity, making it invaluable as an internal standard in mass spectrometry (MS) and liquid chromatography-tandem MS (LC-MS/MS) for quantifying veratric acid in complex matrices . Veratric acid itself is widely distributed in plants, such as Miscanthus sacchariflorus (384.38 ± 5.14 µg/g) and olive oils, where it contributes to chemotaxonomic differentiation . Its deuterated form enhances analytical precision by minimizing isotopic interference during quantification .
Preparation Methods
Synthetic Routes and Reaction Conditions
The synthesis of Veratric Acid-d6 typically involves the deuteration of 3,4-dimethoxybenzoic acid. One common method is the exchange of hydrogen atoms with deuterium using deuterated reagents under specific conditions. For instance, the compound can be synthesized by reacting 3,4-dimethoxybenzoic acid with deuterated methanol (CD3OD) in the presence of a catalyst such as palladium on carbon (Pd/C) under reflux conditions .
Industrial Production Methods
While specific industrial production methods for this compound are not widely documented, the general approach involves large-scale deuteration processes. These processes often utilize deuterium gas (D2) or deuterated solvents in the presence of suitable catalysts to achieve the desired level of deuteration .
Chemical Reactions Analysis
Types of Reactions
Veratric Acid-d6 can undergo various chemical reactions, including:
Oxidation: The compound can be oxidized to form corresponding quinones or other oxidized derivatives.
Reduction: Reduction reactions can convert the carboxylic acid group to an alcohol or aldehyde.
Substitution: The methoxy groups can be substituted with other functional groups through nucleophilic substitution reactions.
Common Reagents and Conditions
Oxidation: Common oxidizing agents include potassium permanganate (KMnO4) and chromium trioxide (CrO3).
Reduction: Reducing agents such as lithium aluminum hydride (LiAlH4) or sodium borohydride (NaBH4) are typically used.
Substitution: Nucleophilic reagents like sodium methoxide (NaOCH3) or sodium ethoxide (NaOEt) can be employed for substitution reactions.
Major Products
The major products formed from these reactions depend on the specific conditions and reagents used. For example, oxidation may yield quinones, while reduction can produce alcohols or aldehydes .
Scientific Research Applications
Veratric Acid-d6 has several applications in scientific research:
Chemistry: Used as a tracer in reaction mechanisms to study the effects of deuterium substitution on reaction rates and pathways.
Biology: Employed in metabolic studies to investigate the impact of deuterium on biological processes and enzyme activities.
Medicine: Utilized in drug development to enhance the stability and reduce the metabolic rate of pharmaceutical compounds.
Industry: Applied in the production of deuterated materials for use in nuclear magnetic resonance (NMR) spectroscopy and other analytical techniques
Mechanism of Action
The mechanism by which Veratric Acid-d6 exerts its effects is primarily through the incorporation of deuterium atoms. Deuterium substitution can alter the compound’s metabolic pathways, leading to changes in its pharmacokinetics and pharmacodynamics. The presence of deuterium can also affect the compound’s interaction with molecular targets, such as enzymes and receptors, by altering bond strengths and reaction kinetics .
Comparison with Similar Compounds
Comparison with Structurally Similar Phenolic Acids
Structural and Functional Analogues
Veratric acid belongs to the methoxy-substituted benzoic acid family. Key analogues include:
Key Differences :
- Substitution Patterns : Veratric acid has two methoxy groups (3,4-OCH₃), whereas vanillic acid has one methoxy and one hydroxyl group. Protocatechuic acid lacks methoxy groups, featuring two hydroxyl groups instead .
- Bioactivity : Veratric acid exhibits antihypertensive effects distinct from salicylic acid’s anti-inflammatory properties. Vanillic acid’s antimicrobial activity is linked to its hydroxyl-methoxy balance .
Metabolic Pathways and Degradation Kinetics
Catabolic Pathways
Veratric acid is metabolized by Nocardia via sequential demethylation to vanillic acid, isovanillic acid, and protocatechuic acid, which enters the β-ketoadipate pathway . In contrast, chlorogenic acid undergoes hydrolysis to caffeic acid and quinic acid, bypassing methoxy group modifications .
Degradation Efficiency
Electrochemical oxidation of veratric acid on boron-doped diamond (BDD) anodes shows pseudo-first-order kinetics (rate constant k = 0.016 min⁻¹), independent of initial concentration (10–50 mg/L). This contrasts with chlorogenic acid, which degrades faster due to its ester linkage susceptibility .
Analytical Performance Comparison
Detection Limits in LC-MS/MS
Compound | LOD (ng/mL) | LOQ (ng/mL) |
---|---|---|
Veratric Acid | 1570 | 3140 |
Caffeic Acid | 1.7 | 3.5 |
Ferulic Acid | 15 | 30 |
Veratric acid’s higher LOD/LOQ compared to caffeic or ferulic acids reflects challenges in ionizing methoxy-rich structures .
Role of Deuterated Forms
Veratric Acid-d6, like Fusidic Acid-d6, serves as an internal standard to correct matrix effects in MS. Its deuterium labeling avoids overlap with non-deuterated analytes, unlike non-labeled vanillic acid, which requires careful chromatographic separation .
Biological Activity
Veratric Acid-d6, also known as 3,4-Bis(trideuteriomethoxy)benzoic acid, is a deuterated derivative of veratric acid, a compound found in various fruits and vegetables. This article delves into the biological activity of this compound, highlighting its mechanisms of action, pharmacological effects, and applications in research and medicine.
Target Interaction
this compound primarily interacts with biological targets by modulating cellular processes. It has been shown to:
- Inhibit Cyclobutane Pyrimidine Dimers (CPDs) : This action reduces DNA damage induced by UV radiation.
- Reduce Glutathione (GSH) Depletion : GSH is a crucial antioxidant in cells; maintaining its levels helps protect against oxidative stress.
- Inhibit Apoptosis : The compound prevents cell death triggered by UVB exposure, thereby promoting cell survival.
Biochemical Pathways
This compound undergoes microbial degradation, leading to the formation of intermediate metabolites such as vanillic acid and protocatechuic acid. These metabolites are incorporated into anabolic pathways, contributing to cellular biomass. Additionally, the compound has been implicated in the regulation of the NF-κB signaling pathway through inhibition of IκB and p65 phosphorylation, which is vital for controlling inflammatory responses.
Pharmacokinetics
This compound is noted for its oral bioactivity. In studies involving hypertensive rats, administration at a dose of 40 mg/kg body weight demonstrated significant improvements in cardiovascular function and oxidative stress markers.
Cardiovascular Effects
A study assessed the effects of Veratric Acid on hypertensive rats induced by Nω-nitro-L-arginine methyl ester hydrochloride (l-NAME). Key findings include:
- Improvement in Cardiac Function : Functional studies using the Langendorff isolated heart system indicated enhanced ventricular performance.
- Reduction in Lipid Peroxidation : Levels of thiobarbituric acid-reactive substances (TBARS) decreased significantly.
- Antioxidant Status : Increased activities of superoxide dismutase (SOD), catalase, and glutathione peroxidase were observed post-treatment .
Parameter | Control Group | l-NAME Group | This compound Group |
---|---|---|---|
TBARS (μmol/L) | 0.5 | 1.5 | 0.8 |
SOD Activity (U/mg protein) | 10 | 5 | 8 |
Catalase Activity (U/mg protein) | 15 | 7 | 12 |
Anti-inflammatory Activity
In vitro studies have shown that this compound possesses anti-inflammatory properties:
- Inhibition of iNOS Expression : In LPS-stimulated RAW264.7 cells, it reduced iNOS levels at concentrations of 100-200 μM.
- Suppression of COX-2 Expression : The compound also decreased COX-2 and PGE2 levels following UVB exposure .
Case Studies
- Hypertensive Rats Study : A controlled experiment demonstrated that this compound significantly normalized cardiovascular function in hypertensive rats over four weeks when administered at a dose of 40 mg/kg .
- UVB-Induced Damage : In cell cultures exposed to UVB radiation, this compound protected HaCaT cells from phototoxicity by reducing apoptosis and promoting cell cycle progression into the S-phase .
Q & A
Basic Research Questions
Q. What are the established methods for synthesizing and characterizing Veratric Acid-d6?
this compound (deuterated veratric acid) is synthesized via isotopic labeling, typically using acid-catalyzed deuterium exchange or deuterated reagents. Characterization requires nuclear magnetic resonance (NMR) spectroscopy to confirm deuterium incorporation at specific positions (e.g., methoxy groups) and liquid chromatography-mass spectrometry (LC-MS) to verify isotopic purity (>98% deuterium). For validation, compare spectral data with non-deuterated veratric acid and use internal standards to ensure reproducibility .
Q. Which analytical techniques are most reliable for quantifying this compound in biological matrices?
LC-MS/MS is the gold standard due to its sensitivity in distinguishing isotopic peaks. Method validation should include calibration curves (1–1000 ng/mL range), spike-and-recovery tests in plasma/tissue homogenates, and stability assessments under varying pH/temperature. Use deuterated internal standards (e.g., this compound itself) to correct for matrix effects .
Q. How do researchers standardize protocols for assessing this compound’s antioxidant activity?
Employ the DPPH (2,2-diphenyl-1-picrylhydrazyl) and ABTS (2,2'-azino-bis(3-ethylbenzothiazoline-6-sulfonic acid)) radical scavenging assays. Prepare this compound in dimethyl sulfoxide (DMSO), test concentrations from 10–200 µg/mL, and measure absorbance at 517 nm (DPPH) or 734 nm (ABTS). Include ascorbic acid as a positive control and calculate IC50 values using nonlinear regression .
Advanced Research Questions
Q. How can researchers resolve contradictions in reported IC50 values for this compound across studies?
Discrepancies often arise from variability in assay conditions (e.g., solvent polarity, incubation time). To address this:
- Perform a meta-analysis of published data, stratifying results by methodology.
- Replicate experiments under controlled conditions (e.g., fixed pH, temperature).
- Use standardized reference materials (e.g., NIST-traceable deuterated compounds) to calibrate assays .
Q. What experimental designs are optimal for evaluating the metabolic stability of this compound in vitro?
Use liver microsomes (human/rat) incubated with this compound (1–50 µM) and NADPH. Quantify parent compound degradation via LC-MS at 0, 15, 30, and 60 minutes. Calculate half-life (t½) using first-order kinetics. Include controls without NADPH to distinguish enzymatic vs. non-enzymatic degradation. Compare results with non-deuterated veratric acid to assess isotope effects .
Q. How can deuterium labeling in this compound influence its pharmacokinetic (PK) profile in vivo?
Deuterium can alter hydrogen-deuterium exchange rates, potentially affecting metabolic pathways. To study this:
- Administer this compound and non-deuterated analogs to animal models (e.g., rats) via IV/oral routes.
- Collect plasma samples at timed intervals and quantify using LC-MS.
- Compare AUC (area under the curve), Cmax, and t½ to assess differences in bioavailability and clearance .
Q. What strategies mitigate isotopic interference when tracking this compound in complex biological systems?
- Use high-resolution mass spectrometry (HRMS) to resolve isotopic clusters from endogenous compounds.
- Apply stable isotope-labeled internal standards (SIL-IS) with distinct deuterium positions.
- Validate methods using spike-in experiments in relevant biological fluids (e.g., urine, serum) .
Q. Methodological Challenges and Solutions
Q. How should researchers design dose-response studies for this compound to ensure statistical rigor?
- Use at least six concentrations spanning three orders of magnitude.
- Replicate experiments ≥3 times to account for biological variability.
- Apply ANOVA with post-hoc tests (e.g., Tukey’s) for multi-group comparisons.
- Report confidence intervals for IC50/EC50 values .
Q. What approaches validate the specificity of this compound in cellular uptake assays?
- Combine LC-MS with fluorescence tagging (if applicable) for dual confirmation.
- Perform competition assays with excess non-deuterated veratric acid to block specific uptake pathways.
- Use CRISPR-edited cell lines lacking putative transporters (e.g., OATPs) to confirm mechanism .
Q. How can researchers address low signal-to-noise ratios in NMR analyses of this compound?
- Use cryoprobes to enhance sensitivity.
- Employ deuterated solvents (e.g., DMSO-d6) to minimize background interference.
- Collect data at high magnetic field strengths (≥600 MHz) and apply advanced processing algorithms (e.g., line broadening <1 Hz) .
Q. Data Interpretation and Contradictions
Q. Why might this compound exhibit reduced antioxidant efficacy in certain in vivo models despite strong in vitro data?
Potential factors include:
- Rapid metabolism or protein binding in vivo.
- Tissue-specific differences in ROS (reactive oxygen species) generation.
- Interactions with endogenous antioxidants (e.g., glutathione).
Solution: Perform tissue distribution studies and measure ROS levels directly via fluorescence probes (e.g., DCFH-DA) .
Q. How can researchers reconcile conflicting data on this compound’s cytotoxicity across cell lines?
Properties
IUPAC Name |
3,4-bis(trideuteriomethoxy)benzoic acid | |
---|---|---|
Source | PubChem | |
URL | https://pubchem.ncbi.nlm.nih.gov | |
Description | Data deposited in or computed by PubChem | |
InChI |
InChI=1S/C9H10O4/c1-12-7-4-3-6(9(10)11)5-8(7)13-2/h3-5H,1-2H3,(H,10,11)/i1D3,2D3 | |
Source | PubChem | |
URL | https://pubchem.ncbi.nlm.nih.gov | |
Description | Data deposited in or computed by PubChem | |
InChI Key |
DAUAQNGYDSHRET-WFGJKAKNSA-N | |
Source | PubChem | |
URL | https://pubchem.ncbi.nlm.nih.gov | |
Description | Data deposited in or computed by PubChem | |
Canonical SMILES |
COC1=C(C=C(C=C1)C(=O)O)OC | |
Source | PubChem | |
URL | https://pubchem.ncbi.nlm.nih.gov | |
Description | Data deposited in or computed by PubChem | |
Isomeric SMILES |
[2H]C([2H])([2H])OC1=C(C=C(C=C1)C(=O)O)OC([2H])([2H])[2H] | |
Source | PubChem | |
URL | https://pubchem.ncbi.nlm.nih.gov | |
Description | Data deposited in or computed by PubChem | |
Molecular Formula |
C9H10O4 | |
Source | PubChem | |
URL | https://pubchem.ncbi.nlm.nih.gov | |
Description | Data deposited in or computed by PubChem | |
Molecular Weight |
188.21 g/mol | |
Source | PubChem | |
URL | https://pubchem.ncbi.nlm.nih.gov | |
Description | Data deposited in or computed by PubChem | |
Disclaimer and Information on In-Vitro Research Products
Please be aware that all articles and product information presented on BenchChem are intended solely for informational purposes. The products available for purchase on BenchChem are specifically designed for in-vitro studies, which are conducted outside of living organisms. In-vitro studies, derived from the Latin term "in glass," involve experiments performed in controlled laboratory settings using cells or tissues. It is important to note that these products are not categorized as medicines or drugs, and they have not received approval from the FDA for the prevention, treatment, or cure of any medical condition, ailment, or disease. We must emphasize that any form of bodily introduction of these products into humans or animals is strictly prohibited by law. It is essential to adhere to these guidelines to ensure compliance with legal and ethical standards in research and experimentation.