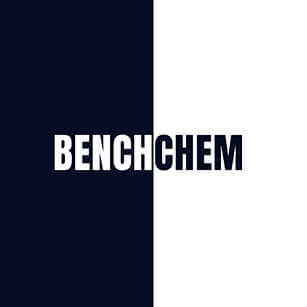
Sulindac Acyl-β-D-Glucuronide
- Click on QUICK INQUIRY to receive a quote from our team of experts.
- With the quality product at a COMPETITIVE price, you can focus more on your research.
Overview
Description
Sulindac Acyl-β-D-Glucuronide is a metabolite of the nonsteroidal anti-inflammatory drug (NSAID) Sulindac. It is formed through the conjugation of Sulindac with β-D-glucuronic acid. This compound is of significant interest due to its role in drug metabolism and potential implications in drug safety and efficacy .
Mechanism of Action
Target of Action
Sulindac Acyl-β-D-Glucuronide is a metabolite of the non-steroidal anti-inflammatory drug (NSAID) Sulindac . Its primary targets are proteins in the body, specifically those that interact with carboxylic acid-containing drugs .
Mode of Action
This compound interacts with its protein targets through a process known as intramolecular transacylation and hydrolysis . This process involves the transfer of an acyl group from the glucuronide to the protein, resulting in the formation of protein adducts . The charged carboxylate ion and neutral hydroxyl group in the glucuronide conjugate are responsible for these interactions .
Biochemical Pathways
The formation of this compound involves the conjugation of α-D-glucuronic acid with the carboxylic acid group of a xenobiotic in the presence of membrane-bound UDP-glucuronosyltransferase (UGT) enzymes . This process is part of the glucuronidation pathway, which is a major phase II metabolic pathway in the body.
Pharmacokinetics
It is known that acyl glucuronides, in general, are predominantly cleared through renal excretion . The molecular weight of this compound is 532.53 , which suggests that it may be subject to renal clearance.
Result of Action
The formation of protein adducts by this compound has been associated with the toxicity of several carboxylic acid-containing drugs .
Action Environment
The action of this compound can be influenced by various environmental factors. For instance, the pH of the environment can affect the rate of intramolecular transacylation and hydrolysis . Additionally, the presence of other substances in the body that can interact with this compound may also influence its action, efficacy, and stability.
Biochemical Analysis
Biochemical Properties
Sulindac Acyl-β-D-Glucuronide is involved in various biochemical reactions. It is formed through the conjugation of α-D-glucuronic acid with the carboxylic acid group of a xenobiotic in the presence of membrane-bound UDP-glucuronosyltransferase (UGT) enzymes . The rate of its degradation via intramolecular transacylation and hydrolysis has been associated with the degree of protein adduct formation .
Cellular Effects
The cellular effects of this compound are complex and multifaceted. It has been implicated in the toxicity of several carboxylic acid-containing drugs . The formation of protein adducts in vivo – and subsequent downstream effects – has been proposed as a mechanism of toxicity for carboxylic acid-containing xenobiotics capable of forming acyl glucuronides .
Molecular Mechanism
At the molecular level, this compound exerts its effects through various mechanisms. It undergoes intramolecular transacylation and hydrolysis reactions . Differences in reactivity were observed for the individual transacylation steps between the compound series . The transacylation reaction was modelled using density functional theory and the calculated activation energy for this reaction showed a close correlation with the degradation rate of the 1-β anomer .
Temporal Effects in Laboratory Settings
In laboratory settings, the effects of this compound change over time. The compound is known to be unstable under physiological conditions
Metabolic Pathways
This compound is involved in the glucuronidation metabolic pathway . This pathway involves the conjugation of α-D-glucuronic acid with the carboxylic acid group of a xenobiotic in the presence of membrane-bound UDP-glucuronosyltransferase (UGT) enzymes .
Transport and Distribution
The transport and distribution of this compound within cells and tissues involve various transport proteins. Uptake transporters, such as organic anion transporters (OATs and OATPs), mediate the uptake of conjugates into the liver and kidney, while efflux transporters, such as multidrug resistance proteins (MRPs) and breast cancer resistance protein (BCRP), mediate expulsion of conjugates into bile, urine and the intestinal lumen .
Preparation Methods
Synthetic Routes and Reaction Conditions
Sulindac Acyl-β-D-Glucuronide is synthesized through the enzymatic conjugation of Sulindac with β-D-glucuronic acid. This reaction is typically catalyzed by UDP-glucuronosyltransferase (UGT) enzymes. The reaction conditions often involve the presence of UDP-glucuronic acid and the UGT enzyme in a suitable buffer solution .
Industrial Production Methods
Industrial production of this compound follows similar principles as the laboratory synthesis but on a larger scale. The process involves the use of bioreactors to facilitate the enzymatic reaction, ensuring optimal conditions for enzyme activity and product yield .
Chemical Reactions Analysis
Types of Reactions
Sulindac Acyl-β-D-Glucuronide undergoes several types of chemical reactions, including:
Hydrolysis: The compound can hydrolyze back to Sulindac and β-D-glucuronic acid.
Transacylation: It can undergo intramolecular transacylation, leading to the formation of isomers.
Oxidation and Reduction: These reactions can modify the functional groups on the Sulindac moiety.
Common Reagents and Conditions
Hydrolysis: Typically occurs in aqueous solutions, often catalyzed by acidic or basic conditions.
Transacylation: Requires the presence of nucleophiles and can be influenced by the pH and temperature of the solution.
Oxidation and Reduction: Common reagents include oxidizing agents like hydrogen peroxide and reducing agents like sodium borohydride.
Major Products Formed
Hydrolysis: Produces Sulindac and β-D-glucuronic acid.
Transacylation: Leads to the formation of positional isomers.
Oxidation and Reduction: Results in various oxidized or reduced forms of Sulindac.
Scientific Research Applications
Sulindac Acyl-β-D-Glucuronide has several applications in scientific research:
Chemistry: Used to study the reactivity and stability of acyl glucuronides.
Biology: Investigated for its role in drug metabolism and potential toxicity.
Medicine: Explored for its implications in drug safety, particularly in understanding adverse drug reactions.
Industry: Utilized in the development of analytical standards and reference materials for drug testing
Comparison with Similar Compounds
Similar Compounds
- Ibuprofen Acyl-β-D-Glucuronide
- Diclofenac Acyl-β-D-Glucuronide
- Naproxen Acyl-β-D-Glucuronide
Uniqueness
Sulindac Acyl-β-D-Glucuronide is unique due to its specific structural features and reactivity profile. Unlike other acyl glucuronides, it has a distinct indene moiety and a fluorine atom, which influence its chemical behavior and biological interactions .
Biological Activity
Sulindac acyl-β-D-glucuronide is a metabolite of sulindac, a nonsteroidal anti-inflammatory drug (NSAID) known for its anti-inflammatory and potential chemopreventive properties. This article will explore the biological activity of this compound, focusing on its pharmacokinetics, mechanisms of action, and implications in therapeutic contexts, supported by relevant research findings and case studies.
1. Overview of Sulindac and Its Metabolites
Sulindac is administered as a prodrug, which is converted into its active forms—sulindac sulfide and sulindac sulfone—through metabolic processes. The acyl-β-D-glucuronide form is a significant metabolite that influences the drug's pharmacological profile.
Key Metabolites:
- Sulindac Sulfide : Exhibits potent anti-inflammatory effects and has been shown to inhibit cyclooxygenase (COX) enzymes.
- Sulindac Sulfone : Less active than sulfide but still plays a role in the drug's overall efficacy.
- This compound : A conjugated form that may enhance solubility and excretion.
The biological activity of this compound primarily involves its role in modulating inflammatory pathways and potential anticancer effects.
Mechanisms Include:
- Inhibition of COX Enzymes : Like other NSAIDs, sulindac metabolites inhibit COX-1 and COX-2, leading to decreased prostaglandin synthesis which is crucial in the inflammatory process.
- Induction of Apoptosis : Studies have shown that sulindac can induce apoptosis in various cancer cell lines, potentially through mitochondrial pathways involving cytochrome c release and activation of caspases .
- Autophagy Modulation : Recent findings suggest that sulindac may induce autophagic processes in neurons, linking it to neurodevelopmental toxicity risks .
3. Pharmacokinetics
The pharmacokinetic profile of this compound indicates that it is well absorbed and undergoes extensive metabolism. The glucuronidation process enhances the compound's water solubility, facilitating renal excretion.
Pharmacokinetic Parameters:
Parameter | Value |
---|---|
Molecular Formula | C26H25FO10S |
Molecular Weight | 485.54 g/mol |
Elimination Half-life | Approximately 8 hours |
Route of Excretion | Renal (urine) |
4. Case Studies and Research Findings
Several studies have highlighted the biological activity of this compound in various contexts:
Case Study 1: Cancer Prevention
A randomized controlled trial involving patients with familial adenomatous polyposis (FAP) demonstrated that treatment with sulindac resulted in a significant reduction in polyp size and number over nine months . The study indicated that both the parent drug and its metabolites contributed to these effects.
Case Study 2: Neurotoxicity Assessment
Research utilizing zebrafish models revealed that exposure to sulindac led to apoptosis in GABAergic neurons, suggesting potential neurodevelopmental risks associated with its use . The study emphasized the importance of understanding the effects of both active metabolites and their glucuronidated forms on neural health.
5. Conclusion
This compound represents an important metabolite in the pharmacological action of sulindac, contributing to its anti-inflammatory and potential anticancer properties. Understanding its biological activity helps clarify the therapeutic benefits and risks associated with sulindac use, particularly regarding long-term safety profiles in vulnerable populations.
Properties
CAS No. |
60018-36-2 |
---|---|
Molecular Formula |
C26H25FO9S |
Molecular Weight |
532.5 g/mol |
IUPAC Name |
(2S,3S,4S,5R,6S)-6-[2-[(3Z)-6-fluoro-2-methyl-3-[(4-methylsulfinylphenyl)methylidene]inden-1-yl]acetyl]oxy-3,4,5-trihydroxyoxane-2-carboxylic acid |
InChI |
InChI=1S/C26H25FO9S/c1-12-17(9-13-3-6-15(7-4-13)37(2)34)16-8-5-14(27)10-19(16)18(12)11-20(28)35-26-23(31)21(29)22(30)24(36-26)25(32)33/h3-10,21-24,26,29-31H,11H2,1-2H3,(H,32,33)/b17-9-/t21-,22-,23+,24-,26+,37?/m0/s1 |
InChI Key |
ZGOXLKUBXKIPQX-AEHOTZTESA-N |
SMILES |
CC1=C(C2=C(C1=CC3=CC=C(C=C3)S(=O)C)C=CC(=C2)F)CC(=O)OC4C(C(C(C(O4)C(=O)O)O)O)O |
Isomeric SMILES |
CC\1=C(C2=C(/C1=C\C3=CC=C(C=C3)S(=O)C)C=CC(=C2)F)CC(=O)O[C@H]4[C@@H]([C@H]([C@@H]([C@H](O4)C(=O)O)O)O)O |
Canonical SMILES |
CC1=C(C2=C(C1=CC3=CC=C(C=C3)S(=O)C)C=CC(=C2)F)CC(=O)OC4C(C(C(C(O4)C(=O)O)O)O)O |
Synonyms |
(Z)-1-[5-Fluoro-2-methyl-1-[[4-(methylsulfinyl)phenyl]methylene]-1H-indene-3-acetate]β-D-Glucopyranuronic Acid; |
Origin of Product |
United States |
Disclaimer and Information on In-Vitro Research Products
Please be aware that all articles and product information presented on BenchChem are intended solely for informational purposes. The products available for purchase on BenchChem are specifically designed for in-vitro studies, which are conducted outside of living organisms. In-vitro studies, derived from the Latin term "in glass," involve experiments performed in controlled laboratory settings using cells or tissues. It is important to note that these products are not categorized as medicines or drugs, and they have not received approval from the FDA for the prevention, treatment, or cure of any medical condition, ailment, or disease. We must emphasize that any form of bodily introduction of these products into humans or animals is strictly prohibited by law. It is essential to adhere to these guidelines to ensure compliance with legal and ethical standards in research and experimentation.