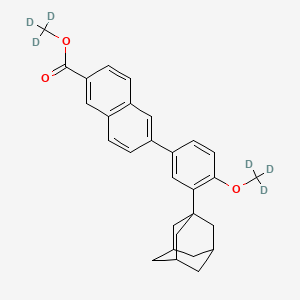
Adapalene-d6 Methyl Ester
Overview
Description
Adapalene-d6 Methyl Ester is a deuterium-labeled derivative of Adapalene, a third-generation topical retinoid. This compound is primarily used in pharmaceutical research as a reference standard and impurity marker. Adapalene itself is widely recognized for its efficacy in treating acne vulgaris due to its comedolytic, anti-inflammatory, and antiproliferative properties .
Mechanism of Action
Target of Action
Adapalene-d6 Methyl Ester, a derivative of Adapalene, primarily targets retinoic acid nuclear receptors . These receptors play a crucial role in regulating gene expression and reducing inflammation .
Mode of Action
This compound interacts with its targets by binding to retinoic acid nuclear receptors . This binding inhibits keratinocyte differentiation, a process found in the outermost layer of the skin, the epidermis . It also exhibits exfoliating and anti-inflammatory effects .
Biochemical Pathways
The compound affects the biochemical pathways related to inflammation and keratinocyte differentiation . By binding to retinoic acid nuclear receptors, it modulates gene expression, leading to reduced inflammation and inhibited keratinocyte differentiation . The downstream effects include the comedolytic (preventing comedones or acne), anti-inflammatory, antiproliferative, and immunomodulatory effects .
Pharmacokinetics
Innovative formulations aim to achieve better bioavailability, increased efficacy, and reduced side effects .
Result of Action
The molecular and cellular effects of this compound’s action include comedolytic, anti-inflammatory, antiproliferative, and immunomodulatory effects . It has been found to be effective in the treatment of several dermatological diseases and photoaging, in addition to its utility in the treatment of acne vulgaris .
Action Environment
New topical combinations include ketoconazole (antifungal), mometasone furoate (anti-inflammatory corticosteroid), nadifloxacin (fluoroquinolone), and alfa and beta hydroxy acids . Combination with oral drugs is a new trend that enhances the properties of topical formulations with this compound .
Biochemical Analysis
Biochemical Properties
Adapalene-d6 Methyl Ester, like its parent compound Adapalene, binds to retinoic acid nuclear receptors . The known effects of Adapalene include comedolytic, anti-inflammatory, antiproliferative, and immunomodulatory actions . These interactions with various biomolecules play a crucial role in its biochemical reactions.
Cellular Effects
This compound influences cell function by impacting cell signaling pathways, gene expression, and cellular metabolism . For instance, it has been found to inhibit human polymorphonuclear neutrophil leukocyte activation and lipoxygenase enzymes .
Molecular Mechanism
The molecular mechanism of action of this compound involves binding interactions with biomolecules, enzyme inhibition or activation, and changes in gene expression . Adapalene binds directly to retinoic acid receptors (RARs); the adapalene-RAR complex forms heterodimers with retinoid X receptors (RXRs), which can bind DNA and modulate transcription .
Temporal Effects in Laboratory Settings
In laboratory settings, the effects of this compound change over time . It exhibits an intense native fluorescence at 389 nm after excitation at 312 nm using borate buffer (pH 7.0)/ethanol system . This property is used for routine analysis of this compound in its gel and ideally suited to the in vitro diffusion test .
Dosage Effects in Animal Models
The effects of this compound vary with different dosages in animal models . For instance, in a rabbit ear acne model, the effects of this compound were equivalent to those of adapalene gel without local side effects .
Metabolic Pathways
It is known that this compound, like Adapalene, binds to retinoic acid nuclear receptors, which suggests it may be involved in the retinoic acid metabolic pathway .
Preparation Methods
Synthetic Routes and Reaction Conditions
The synthesis of Adapalene-d6 Methyl Ester involves several key steps:
Coupling: The zinc derivative of 2-(1-adamantyl)-4-bromoanisole is coupled with methyl-6-bromo-2-naphthenoate using a nickel catalyst.
Industrial Production Methods
Industrial production of this compound follows similar synthetic routes but is optimized for higher yields and purity. The process involves large-scale reactors and stringent quality control measures to ensure the consistency and reliability of the final product .
Chemical Reactions Analysis
Adapalene-d6 Methyl Ester undergoes various chemical reactions, including:
Oxidation: This compound can be oxidized under specific conditions to form corresponding oxidized derivatives.
Reduction: Reduction reactions can convert this compound into its reduced forms.
Substitution: The compound can undergo substitution reactions, particularly in the presence of nucleophiles or electrophiles.
Common reagents used in these reactions include oxidizing agents like potassium permanganate, reducing agents like lithium aluminum hydride, and various nucleophiles and electrophiles depending on the desired substitution . The major products formed from these reactions are typically derivatives of this compound with modified functional groups.
Scientific Research Applications
Adapalene-d6 Methyl Ester is extensively used in scientific research, particularly in:
Pharmaceutical Research: As a reference standard and impurity marker in the development and quality control of Adapalene-based formulations.
Biological Studies: Investigating the metabolic pathways and biological effects of Adapalene and its derivatives.
Medical Research: Exploring the potential therapeutic applications of Adapalene in treating various dermatological conditions and other diseases.
Industrial Applications: Used in the synthesis of high-purity Adapalene for commercial pharmaceutical products.
Comparison with Similar Compounds
Adapalene-d6 Methyl Ester is unique due to its deuterium labeling, which provides enhanced stability and allows for precise tracking in metabolic studies. Similar compounds include:
Adapalene: The non-labeled version used widely in acne treatment.
Tazarotene: Another third-generation retinoid with similar therapeutic applications but different molecular targets.
This compound stands out due to its specific use in research and its enhanced stability provided by deuterium labeling .
Properties
IUPAC Name |
trideuteriomethyl 6-[3-(1-adamantyl)-4-(trideuteriomethoxy)phenyl]naphthalene-2-carboxylate | |
---|---|---|
Source | PubChem | |
URL | https://pubchem.ncbi.nlm.nih.gov | |
Description | Data deposited in or computed by PubChem | |
InChI |
InChI=1S/C29H30O3/c1-31-27-8-7-24(14-26(27)29-15-18-9-19(16-29)11-20(10-18)17-29)22-3-4-23-13-25(28(30)32-2)6-5-21(23)12-22/h3-8,12-14,18-20H,9-11,15-17H2,1-2H3/i1D3,2D3 | |
Source | PubChem | |
URL | https://pubchem.ncbi.nlm.nih.gov | |
Description | Data deposited in or computed by PubChem | |
InChI Key |
PGXNMQBGOVUZNC-WFGJKAKNSA-N | |
Source | PubChem | |
URL | https://pubchem.ncbi.nlm.nih.gov | |
Description | Data deposited in or computed by PubChem | |
Canonical SMILES |
COC1=C(C=C(C=C1)C2=CC3=C(C=C2)C=C(C=C3)C(=O)OC)C45CC6CC(C4)CC(C6)C5 | |
Source | PubChem | |
URL | https://pubchem.ncbi.nlm.nih.gov | |
Description | Data deposited in or computed by PubChem | |
Isomeric SMILES |
[2H]C([2H])([2H])OC1=C(C=C(C=C1)C2=CC3=C(C=C2)C=C(C=C3)C(=O)OC([2H])([2H])[2H])C45CC6CC(C4)CC(C6)C5 | |
Source | PubChem | |
URL | https://pubchem.ncbi.nlm.nih.gov | |
Description | Data deposited in or computed by PubChem | |
Molecular Formula |
C29H30O3 | |
Source | PubChem | |
URL | https://pubchem.ncbi.nlm.nih.gov | |
Description | Data deposited in or computed by PubChem | |
Molecular Weight |
432.6 g/mol | |
Source | PubChem | |
URL | https://pubchem.ncbi.nlm.nih.gov | |
Description | Data deposited in or computed by PubChem | |
Retrosynthesis Analysis
AI-Powered Synthesis Planning: Our tool employs the Template_relevance Pistachio, Template_relevance Bkms_metabolic, Template_relevance Pistachio_ringbreaker, Template_relevance Reaxys, Template_relevance Reaxys_biocatalysis model, leveraging a vast database of chemical reactions to predict feasible synthetic routes.
One-Step Synthesis Focus: Specifically designed for one-step synthesis, it provides concise and direct routes for your target compounds, streamlining the synthesis process.
Accurate Predictions: Utilizing the extensive PISTACHIO, BKMS_METABOLIC, PISTACHIO_RINGBREAKER, REAXYS, REAXYS_BIOCATALYSIS database, our tool offers high-accuracy predictions, reflecting the latest in chemical research and data.
Strategy Settings
Precursor scoring | Relevance Heuristic |
---|---|
Min. plausibility | 0.01 |
Model | Template_relevance |
Template Set | Pistachio/Bkms_metabolic/Pistachio_ringbreaker/Reaxys/Reaxys_biocatalysis |
Top-N result to add to graph | 6 |
Feasible Synthetic Routes
Disclaimer and Information on In-Vitro Research Products
Please be aware that all articles and product information presented on BenchChem are intended solely for informational purposes. The products available for purchase on BenchChem are specifically designed for in-vitro studies, which are conducted outside of living organisms. In-vitro studies, derived from the Latin term "in glass," involve experiments performed in controlled laboratory settings using cells or tissues. It is important to note that these products are not categorized as medicines or drugs, and they have not received approval from the FDA for the prevention, treatment, or cure of any medical condition, ailment, or disease. We must emphasize that any form of bodily introduction of these products into humans or animals is strictly prohibited by law. It is essential to adhere to these guidelines to ensure compliance with legal and ethical standards in research and experimentation.