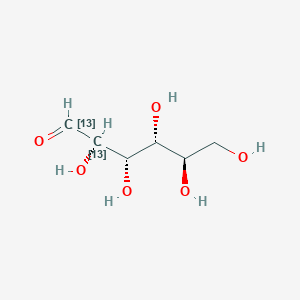
D-GLUCOSE-1,2-13C2
Overview
Description
D-Glucose-1,2-13C2 is a stable isotope-labeled compound of D-Glucose. It is specifically labeled with carbon-13 at the first and second carbon positions. This compound is used extensively in metabolic studies due to its ability to trace the metabolic pathways of glucose in biological systems .
Mechanism of Action
Target of Action
D-Glucose-13C2-4, also known as D-Glucose-1,2-13C2, is a labeled form of D-Glucose . D-Glucose is a monosaccharide and an essential carbohydrate in biology . It serves as a critical component of general metabolism and acts as a key signaling molecule in relation to both cellular metabolic status and biotic and abiotic stress response . It has been used in the study of dynamic metabolic profiling of pancreatic tumors .
Mode of Action
D-Glucose-13C2-4 interacts with its targets in a similar way to D-Glucose. It is incorporated into drug molecules, largely as tracers for quantitation during the drug development process . Deuteration has gained attention because of its potential to affect the pharmacokinetic and metabolic profiles of drugs .
Biochemical Pathways
D-Glucose-13C2-4 is involved in several biochemical pathways. One of the primary pathways is glycolysis, where glucose is converted to pyruvate, yielding ATP and NADH . This pathway is also known as the Embden-Meyerhof-Parnas pathway . D-Glucose-13C2-4 can also be used to study the nonoxidative pentose phosphate pathway using a single-tracer method .
Pharmacokinetics
The pharmacokinetics of D-Glucose-13C2-4 is similar to that of D-Glucose. Stable heavy isotopes of hydrogen, carbon, and other elements have been incorporated into drug molecules, largely as tracers for quantitation during the drug development process . Deuteration has gained attention because of its potential to affect the pharmacokinetic and metabolic profiles of drugs .
Result of Action
The result of D-Glucose-13C2-4 action is primarily the production of energy in the form of ATP and NADH through the process of glycolysis . It also serves as a critical signaling molecule in relation to both cellular metabolic status and biotic and abiotic stress response .
Action Environment
The action of D-Glucose-13C2-4 can be influenced by various environmental factors. For instance, the presence or absence of oxygen can determine whether cells undergo aerobic respiration (with oxygen) or fermentation (without oxygen) . Furthermore, the efficacy and stability of D-Glucose-13C2-4 can be affected by factors such as temperature, pH, and the presence of other molecules in the environment.
Biochemical Analysis
Biochemical Properties
D-Glucose-13C2-4 plays a significant role in biochemical reactions. It interacts with various enzymes, proteins, and other biomolecules. For instance, it is a critical participant in inflammation-related signaling pathways . The enzyme glucose oxidase has been used in the production of D-gluconic acid, which is a reactant of undoubtable interest in different industrial areas .
Cellular Effects
D-Glucose-13C2-4 has profound effects on various types of cells and cellular processes. It influences cell function, including any impact on cell signaling pathways, gene expression, and cellular metabolism . For example, it serves as a critical signaling molecule in relation to both cellular metabolic status and biotic and abiotic stress response .
Molecular Mechanism
The mechanism of action of D-Glucose-13C2-4 is complex and involves interactions at the molecular level. It exerts its effects through binding interactions with biomolecules, enzyme inhibition or activation, and changes in gene expression . For instance, it binds to transforming growth factor β-activated kinase 1 (TAK1) and inhibits K63 ubiquitination and phosphorylation of TAK1, thereby dampening downstream inflammatory pathways and promoting insulin signaling pathways .
Temporal Effects in Laboratory Settings
The effects of D-Glucose-13C2-4 change over time in laboratory settings. Information on the product’s stability, degradation, and any long-term effects on cellular function observed in in vitro or in vivo studies is crucial . For instance, the distinct 13C labeling patterns of metabolites from D-Glucose-13C2-4 tracing in vivo and in vitro have been reported .
Dosage Effects in Animal Models
The effects of D-Glucose-13C2-4 vary with different dosages in animal models . This includes any threshold effects observed in these studies, as well as any toxic or adverse effects at high doses.
Metabolic Pathways
D-Glucose-13C2-4 is involved in various metabolic pathways, including glycolysis, the pentose phosphate pathway, and the tricarboxylic acid (TCA) cycle . It interacts with enzymes or cofactors and can affect metabolic flux or metabolite levels.
Transport and Distribution
D-Glucose-13C2-4 is transported and distributed within cells and tissues . This includes any transporters or binding proteins that it interacts with, as well as any effects on its localization or accumulation.
Subcellular Localization
The subcellular localization of D-Glucose-13C2-4 and any effects on its activity or function are important aspects of its biochemical profile . This could include any targeting signals or post-translational modifications that direct it to specific compartments or organelles.
Preparation Methods
Synthetic Routes and Reaction Conditions: The preparation of D-Glucose-1,2-13C2 involves the incorporation of carbon-13 isotopes into the glucose molecule. This can be achieved through chemical synthesis or biosynthetic methods using carbon-13 labeled precursors. The reaction conditions typically involve controlled environments to ensure the precise incorporation of the isotopes .
Industrial Production Methods: Industrial production of this compound is carried out using advanced techniques to ensure high purity and isotopic enrichment. The process involves the use of carbon-13 labeled substrates in fermentation or chemical synthesis, followed by purification steps to isolate the labeled glucose .
Chemical Reactions Analysis
Types of Reactions: D-Glucose-1,2-13C2 undergoes various chemical reactions similar to those of natural glucose. These include:
Oxidation: Conversion to gluconic acid or glucuronic acid.
Reduction: Formation of sorbitol.
Substitution: Formation of glucose derivatives through reactions with different reagents.
Common Reagents and Conditions:
Oxidation: Reagents like nitric acid or bromine water under acidic conditions.
Reduction: Catalytic hydrogenation using hydrogen gas and a metal catalyst.
Substitution: Various reagents depending on the desired derivative, such as acetic anhydride for acetylation.
Major Products:
Oxidation: Gluconic acid, glucuronic acid.
Reduction: Sorbitol.
Substitution: Acetylated glucose derivatives.
Scientific Research Applications
D-Glucose-1,2-13C2 is widely used in scientific research, including:
Chemistry: Studying the metabolic pathways of glucose and its derivatives.
Biology: Investigating glucose metabolism in cells and tissues.
Medicine: Researching metabolic disorders and cancer metabolism.
Industry: Used in the production of labeled compounds for research and diagnostic purposes.
Comparison with Similar Compounds
- D-Glucose-13C6
- D-Glucose-6-13C
- D-Glucose-1-13C
- D-Glucose-1,2,3-13C3
Comparison: D-Glucose-1,2-13C2 is unique due to its specific labeling at the first and second carbon positions, which provides detailed insights into the early steps of glucose metabolism.
Properties
IUPAC Name |
(2R,3S,4R,5R)-2,3,4,5,6-pentahydroxy(1,2-13C2)hexanal | |
---|---|---|
Source | PubChem | |
URL | https://pubchem.ncbi.nlm.nih.gov | |
Description | Data deposited in or computed by PubChem | |
InChI |
InChI=1S/C6H12O6/c7-1-3(9)5(11)6(12)4(10)2-8/h1,3-6,8-12H,2H2/t3-,4+,5+,6+/m0/s1/i1+1,3+1 | |
Source | PubChem | |
URL | https://pubchem.ncbi.nlm.nih.gov | |
Description | Data deposited in or computed by PubChem | |
InChI Key |
GZCGUPFRVQAUEE-SZEFTZBMSA-N | |
Source | PubChem | |
URL | https://pubchem.ncbi.nlm.nih.gov | |
Description | Data deposited in or computed by PubChem | |
Canonical SMILES |
C(C(C(C(C(C=O)O)O)O)O)O | |
Source | PubChem | |
URL | https://pubchem.ncbi.nlm.nih.gov | |
Description | Data deposited in or computed by PubChem | |
Isomeric SMILES |
C([C@H]([C@H]([C@@H]([13C@H]([13CH]=O)O)O)O)O)O | |
Source | PubChem | |
URL | https://pubchem.ncbi.nlm.nih.gov | |
Description | Data deposited in or computed by PubChem | |
Molecular Formula |
C6H12O6 | |
Source | PubChem | |
URL | https://pubchem.ncbi.nlm.nih.gov | |
Description | Data deposited in or computed by PubChem | |
DSSTOX Substance ID |
DTXSID10745859 | |
Record name | D-(1,2-~13~C_2_)-arabino-Hexose | |
Source | EPA DSSTox | |
URL | https://comptox.epa.gov/dashboard/DTXSID10745859 | |
Description | DSSTox provides a high quality public chemistry resource for supporting improved predictive toxicology. | |
Molecular Weight |
182.14 g/mol | |
Source | PubChem | |
URL | https://pubchem.ncbi.nlm.nih.gov | |
Description | Data deposited in or computed by PubChem | |
CAS No. |
138079-87-5 | |
Record name | D-(1,2-~13~C_2_)-arabino-Hexose | |
Source | EPA DSSTox | |
URL | https://comptox.epa.gov/dashboard/DTXSID10745859 | |
Description | DSSTox provides a high quality public chemistry resource for supporting improved predictive toxicology. | |
A: D-Glucose-1,2-13C2 is a stable isotope-labeled form of glucose. Researchers used this compound to trace the metabolic fate of glucose within pancreatic cancer cells treated with ellagic acid and lycopene. [] By analyzing how the labeled glucose was metabolized, the researchers could determine the effect of these phytochemicals on glucose-6-phosphate dehydrogenase (G6PD) activity. G6PD is a key enzyme in the pentose phosphate pathway, which plays a crucial role in DNA synthesis. [] The study found that ellagic acid and lycopene synergistically inhibited G6PD activity, potentially contributing to their anti-proliferative effects in pancreatic cancer cells. []
Disclaimer and Information on In-Vitro Research Products
Please be aware that all articles and product information presented on BenchChem are intended solely for informational purposes. The products available for purchase on BenchChem are specifically designed for in-vitro studies, which are conducted outside of living organisms. In-vitro studies, derived from the Latin term "in glass," involve experiments performed in controlled laboratory settings using cells or tissues. It is important to note that these products are not categorized as medicines or drugs, and they have not received approval from the FDA for the prevention, treatment, or cure of any medical condition, ailment, or disease. We must emphasize that any form of bodily introduction of these products into humans or animals is strictly prohibited by law. It is essential to adhere to these guidelines to ensure compliance with legal and ethical standards in research and experimentation.