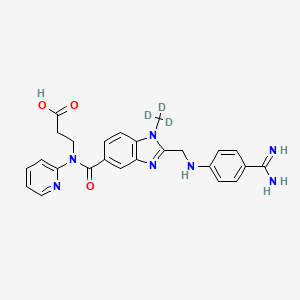
Dabigatran-d3
Overview
Description
Dabigatran-d3 is a deuterium-labeled variant of dabigatran, an oral anticoagulant from the direct thrombin inhibitor class. Dabigatran is widely used to prevent strokes and the formation of blood clots in patients with non-valvular atrial fibrillation and other thromboembolic disorders . The deuterium labeling in this compound enhances its stability and allows for precise quantification in various analytical methods, making it a valuable tool in clinical and pharmaceutical research .
Biochemical Analysis
Biochemical Properties
Dabigatran-d3, like its parent compound dabigatran, inhibits thrombin, a key enzyme in the coagulation cascade . Thrombin plays a crucial role in blood clotting by converting fibrinogen into fibrin. By inhibiting thrombin, this compound prevents this conversion, thereby exerting its anticoagulant effect .
Cellular Effects
This compound, through its inhibition of thrombin, can impact various cellular processes. For instance, it can affect cell proliferation and adhesion, angiogenesis, and invasion, particularly in the context of cancer cells
Molecular Mechanism
This compound acts by binding to the active site of the thrombin molecule, thereby inhibiting its activity . This binding is competitive and reversible . The inhibition of thrombin prevents the conversion of fibrinogen to fibrin, impairing the clotting process and acting as an anticoagulant .
Temporal Effects in Laboratory Settings
Studies on dabigatran have shown that it has predictable anticoagulant effects, does not undergo CYP 450 metabolism, and has few drug–drug and drug–food interactions .
Dosage Effects in Animal Models
The effects of this compound at different dosages in animal models have not been extensively studied. Studies on dabigatran have shown that it has a predictable pharmacokinetic profile, with a clearance of 0.0453 L/min/70 kg, and a central volume of distribution of 2.94 L/70 kg .
Metabolic Pathways
This compound is likely to be involved in similar metabolic pathways as dabigatran. Dabigatran is metabolized by esterases and is a substrate of P-glycoprotein (P-gp) and uridine 5ʹ-diphospho (UDP)-glucuronosyltransferases (UGTs) .
Transport and Distribution
This compound, like dabigatran, is likely to be transported and distributed within cells and tissues via P-gp, a protein that pumps foreign substances out of cells
Preparation Methods
Synthetic Routes and Reaction Conditions: The synthesis of Dabigatran-d3 involves multiple steps, starting from deuterium-labeled bromobenzene. The process includes nitration, cyanation, Pinner reaction, esterification, reduction, and alkylation . The final product is obtained through the condensation of deuterium-labeled O-n-hexylcarbamate derivative with deuterium-labeled benzimidazole .
Industrial Production Methods: Industrial production of this compound follows similar synthetic routes but on a larger scale. The process is optimized for high yield and purity, ensuring that the final product meets stringent quality standards required for pharmaceutical applications .
Chemical Reactions Analysis
Types of Reactions: Dabigatran-d3 undergoes various chemical reactions, including:
Reduction: Involves the removal of oxygen atoms or the addition of hydrogen atoms.
Substitution: Involves the replacement of one atom or group of atoms with another.
Common Reagents and Conditions:
Oxidation: Common reagents include potassium permanganate and hydrogen peroxide.
Reduction: Common reagents include lithium aluminum hydride and sodium borohydride.
Substitution: Common reagents include halogens and nucleophiles under acidic or basic conditions.
Major Products Formed: The major products formed from these reactions depend on the specific conditions and reagents used. For example, oxidation may yield carboxylic acids, while reduction may yield alcohols .
Scientific Research Applications
Dabigatran-d3 has a wide range of scientific research applications, including:
Chemistry: Used as a reference standard in analytical chemistry for the quantification of dabigatran in various samples.
Biology: Used in studies to understand the metabolism and pharmacokinetics of dabigatran.
Medicine: Used in clinical research to develop and validate new anticoagulant therapies.
Industry: Used in the pharmaceutical industry for quality control and regulatory compliance.
Mechanism of Action
Dabigatran-d3, like dabigatran, is a direct thrombin inhibitor. It works by binding to the active site of thrombin, a serine protease that converts fibrinogen to fibrin during the coagulation cascade . By inhibiting thrombin, this compound prevents the formation of blood clots, thereby reducing the risk of stroke and other thromboembolic events .
Comparison with Similar Compounds
Apixaban: Another direct oral anticoagulant that inhibits factor Xa.
Rivaroxaban: A direct oral anticoagulant that also inhibits factor Xa.
Edoxaban: A direct oral anticoagulant that inhibits factor Xa.
Comparison:
Mechanism of Action: While Dabigatran-d3 inhibits thrombin, the other compounds inhibit factor Xa.
Pharmacokinetics: this compound has a predictable pharmacokinetic profile and does not require frequent monitoring, similar to the other direct oral anticoagulants.
Unique Features: The deuterium labeling in this compound enhances its stability and allows for precise quantification, making it unique among anticoagulants.
Properties
IUPAC Name |
3-[[2-[(4-carbamimidoylanilino)methyl]-1-(trideuteriomethyl)benzimidazole-5-carbonyl]-pyridin-2-ylamino]propanoic acid | |
---|---|---|
Source | PubChem | |
URL | https://pubchem.ncbi.nlm.nih.gov | |
Description | Data deposited in or computed by PubChem | |
InChI |
InChI=1S/C25H25N7O3/c1-31-20-10-7-17(25(35)32(13-11-23(33)34)21-4-2-3-12-28-21)14-19(20)30-22(31)15-29-18-8-5-16(6-9-18)24(26)27/h2-10,12,14,29H,11,13,15H2,1H3,(H3,26,27)(H,33,34)/i1D3 | |
Source | PubChem | |
URL | https://pubchem.ncbi.nlm.nih.gov | |
Description | Data deposited in or computed by PubChem | |
InChI Key |
YBSJFWOBGCMAKL-FIBGUPNXSA-N | |
Source | PubChem | |
URL | https://pubchem.ncbi.nlm.nih.gov | |
Description | Data deposited in or computed by PubChem | |
Canonical SMILES |
CN1C2=C(C=C(C=C2)C(=O)N(CCC(=O)O)C3=CC=CC=N3)N=C1CNC4=CC=C(C=C4)C(=N)N | |
Source | PubChem | |
URL | https://pubchem.ncbi.nlm.nih.gov | |
Description | Data deposited in or computed by PubChem | |
Isomeric SMILES |
[2H]C([2H])([2H])N1C2=C(C=C(C=C2)C(=O)N(CCC(=O)O)C3=CC=CC=N3)N=C1CNC4=CC=C(C=C4)C(=N)N | |
Source | PubChem | |
URL | https://pubchem.ncbi.nlm.nih.gov | |
Description | Data deposited in or computed by PubChem | |
Molecular Formula |
C25H25N7O3 | |
Source | PubChem | |
URL | https://pubchem.ncbi.nlm.nih.gov | |
Description | Data deposited in or computed by PubChem | |
DSSTOX Substance ID |
DTXSID70747410 | |
Record name | N-{2-[(4-Carbamimidoylanilino)methyl]-1-(~2~H_3_)methyl-1H-benzimidazole-5-carbonyl}-N-pyridin-2-yl-beta-alanine | |
Source | EPA DSSTox | |
URL | https://comptox.epa.gov/dashboard/DTXSID70747410 | |
Description | DSSTox provides a high quality public chemistry resource for supporting improved predictive toxicology. | |
Molecular Weight |
474.5 g/mol | |
Source | PubChem | |
URL | https://pubchem.ncbi.nlm.nih.gov | |
Description | Data deposited in or computed by PubChem | |
CAS No. |
1246817-44-6 | |
Record name | N-{2-[(4-Carbamimidoylanilino)methyl]-1-(~2~H_3_)methyl-1H-benzimidazole-5-carbonyl}-N-pyridin-2-yl-beta-alanine | |
Source | EPA DSSTox | |
URL | https://comptox.epa.gov/dashboard/DTXSID70747410 | |
Description | DSSTox provides a high quality public chemistry resource for supporting improved predictive toxicology. | |
Synthesis routes and methods I
Procedure details
Synthesis routes and methods II
Procedure details
Q1: What is the role of Dabigatran-d3 in quantifying dabigatran levels?
A1: this compound is a deuterated form of dabigatran used as an internal standard (IS) in mass spectrometry-based analytical methods. [, ] These methods are essential for accurately determining the concentration of free dabigatran in biological samples, such as plasma.
Q2: How does the use of this compound as an IS improve the accuracy of dabigatran quantification?
A2: Using an IS like this compound offers several advantages:
- Compensation for variations: It corrects for potential sample loss during preparation and variation in ionization efficiency during analysis. [, ]
- Improved accuracy: By comparing the signal of dabigatran with the signal of the known concentration of this compound, researchers can accurately calculate the dabigatran concentration in the sample. [, ]
- Enhanced reliability: This approach ensures the reliability and reproducibility of the measurements, which is crucial for pharmacokinetic studies and therapeutic drug monitoring of dabigatran. [, ]
Q3: Can you give an example of how this compound was used in a research setting?
A3: In a study examining the bioequivalence of generic and brand name dabigatran etexilate, researchers utilized a UPLC-MS/MS method with this compound as the IS. [] This allowed them to accurately quantify free dabigatran in patient plasma samples and compare the pharmacokinetic profiles of the two formulations. []
- Development and Validation of UPLC-MS/MS Method for Quantifying of Free and Total Dabigatran in Human Plasma: An Application for a Bioequivalence Study.
- Comparison of calibrated dilute thrombin time and aPTT tests with LC-MS/MS for the therapeutic monitoring of patients treated with dabigatran etexilate
Disclaimer and Information on In-Vitro Research Products
Please be aware that all articles and product information presented on BenchChem are intended solely for informational purposes. The products available for purchase on BenchChem are specifically designed for in-vitro studies, which are conducted outside of living organisms. In-vitro studies, derived from the Latin term "in glass," involve experiments performed in controlled laboratory settings using cells or tissues. It is important to note that these products are not categorized as medicines or drugs, and they have not received approval from the FDA for the prevention, treatment, or cure of any medical condition, ailment, or disease. We must emphasize that any form of bodily introduction of these products into humans or animals is strictly prohibited by law. It is essential to adhere to these guidelines to ensure compliance with legal and ethical standards in research and experimentation.