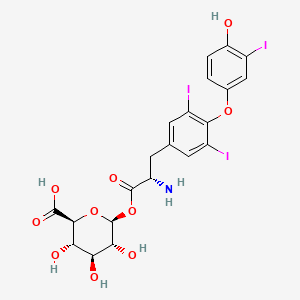
T3 Acyl Glucuronide
Overview
Description
T3 Acyl Glucuronide is a metabolite formed through the conjugation of α-D-glucuronic acid with the carboxylic acid group of a xenobiotic. This process is facilitated by membrane-bound UDP-glucuronosyltransferase enzymes. Acyl glucuronides, including this compound, are known for their potential hepatotoxicity and their role in drug metabolism and clearance .
Preparation Methods
Synthetic Routes and Reaction Conditions: The synthesis of T3 Acyl Glucuronide involves the conjugation of α-D-glucuronic acid with the carboxylic acid group of the parent compound. This reaction is typically catalyzed by UDP-glucuronosyltransferase enzymes. The reaction conditions often include the presence of UDP-glucuronic acid as a cofactor and a suitable buffer system to maintain the pH .
Industrial Production Methods: Industrial production of this compound follows similar principles but on a larger scale. The process involves the use of recombinant UDP-glucuronosyltransferase enzymes and optimized reaction conditions to maximize yield and purity. The product is then purified using chromatographic techniques .
Chemical Reactions Analysis
Types of Reactions: T3 Acyl Glucuronide undergoes several types of reactions, including:
Transacylation: This reaction involves the transfer of the acyl group to nucleophilic centers on proteins or other macromolecules.
Acyl Migration: The acyl group can migrate to different positions on the glucuronic acid moiety, forming various isomers.
Common Reagents and Conditions:
Hydrolysis: Typically occurs under acidic or basic conditions.
Transacylation: Requires nucleophilic reagents such as amino acids or proteins.
Acyl Migration: Occurs spontaneously under physiological conditions.
Major Products:
Hydrolysis: Parent carboxylic acid and glucuronic acid.
Transacylation: Protein or macromolecule adducts.
Acyl Migration: Various positional isomers of the glucuronide.
Scientific Research Applications
T3 Acyl Glucuronide has several applications in scientific research:
Chemistry: Used to study the reactivity and stability of acyl glucuronides and their role in drug metabolism.
Biology: Investigated for its interactions with proteins and potential to form protein adducts.
Medicine: Studied for its role in drug-induced toxicities and its potential as a biomarker for drug metabolism.
Mechanism of Action
T3 Acyl Glucuronide exerts its effects primarily through its reactivity with nucleophilic centers on proteins and other macromolecules. This reactivity can lead to the formation of protein adducts, which may trigger immune responses or other toxicological effects. The molecular targets include amino acid residues such as lysine, cysteine, and serine .
Comparison with Similar Compounds
- Phenylacetic Acid Acyl Glucuronide
- α-Methyl Phenylacetic Acid Acyl Glucuronide
- α,α′-Dimethyl Phenylacetic Acid Acyl Glucuronide
Comparison: T3 Acyl Glucuronide is unique in its specific reactivity and stability profile compared to other acyl glucuronides. The differences in reactivity are attributed to the structural variations in the parent compounds and the resulting glucuronides .
Biological Activity
T3 Acyl Glucuronide is a metabolite derived from the glucuronidation of triiodothyronine (T3), a thyroid hormone crucial for various physiological processes. Understanding the biological activity of this compound is essential for assessing its pharmacokinetics, potential toxicity, and therapeutic implications.
Overview of Glucuronidation
Glucuronidation is a phase II metabolic reaction where glucuronic acid is conjugated to a substrate, enhancing its solubility and facilitating excretion. This process is predominantly catalyzed by UDP-glucuronosyltransferases (UGTs). The glucuronidation of thyroid hormones, particularly T3 and thyroxine (T4), has been shown to involve several UGT isoforms, with varying activity across species.
Enzymatic Activity and Isoform Involvement
Research indicates that T3 undergoes both phenolic and acyl glucuronidation , with specific UGT isoforms playing critical roles.
Key Findings on UGT Isoforms:
- UGT1A3 : Major isoform for both T3 and T4 acyl glucuronides.
- UGT1A8 and UGT1A10 : Significant contributors to the formation of T3 phenolic glucuronides.
- UGT1A1 : Minor involvement in the acyl glucuronidation of T3 .
The relative activity of these isoforms can differ significantly between species. For instance, human liver microsomes show a higher capacity for forming acyl glucuronides compared to those from rats or mice .
Comparative Enzymatic Activity Table
UGT Isoform | Phenolic Glucuronidation Activity (T3) | Acyl Glucuronidation Activity (T3) |
---|---|---|
UGT1A1 | Low | Minor |
UGT1A3 | Moderate | Major |
UGT1A8 | High | Moderate |
UGT1A10 | High | Moderate |
UGT2B4 | Low | Minor |
Biological Implications
The biological activity of this compound can influence several physiological processes:
- Metabolism and Excretion : The formation of acyl glucuronides enhances the elimination of T3 from the body. These metabolites are generally more stable than their parent compounds, which can affect the duration of action of T3 in systemic circulation .
- Potential Toxicity : Acyl glucuronides can undergo transacylation, potentially leading to protein adduct formation. This pathway raises concerns about their reactivity and possible toxic effects, particularly in cases where they may interact with cellular proteins .
Case Studies
Several studies have explored the biological activity of this compound:
- In Vitro Studies : Research using liver microsomes demonstrated that while phenolic glucuronidation predominates in certain species, acyl glucuronidation was notably higher in humans, suggesting a unique metabolic profile that may influence therapeutic outcomes .
- Reactive Intermediate Studies : Investigations into the reactivity of acyl glucuronides revealed that they could form protein adducts through nucleophilic displacement. This finding underscores the importance of understanding the metabolic fate of T3 and its derivatives in drug development and safety assessments .
Properties
IUPAC Name |
(2S,3S,4S,5R,6S)-6-[(2S)-2-amino-3-[4-(4-hydroxy-3-iodophenoxy)-3,5-diiodophenyl]propanoyl]oxy-3,4,5-trihydroxyoxane-2-carboxylic acid | |
---|---|---|
Source | PubChem | |
URL | https://pubchem.ncbi.nlm.nih.gov | |
Description | Data deposited in or computed by PubChem | |
InChI |
InChI=1S/C21H20I3NO10/c22-9-6-8(1-2-13(9)26)33-17-10(23)3-7(4-11(17)24)5-12(25)20(32)35-21-16(29)14(27)15(28)18(34-21)19(30)31/h1-4,6,12,14-16,18,21,26-29H,5,25H2,(H,30,31)/t12-,14-,15-,16+,18-,21-/m0/s1 | |
Source | PubChem | |
URL | https://pubchem.ncbi.nlm.nih.gov | |
Description | Data deposited in or computed by PubChem | |
InChI Key |
LQMBVWCQWFEPFK-DKBYMCRTSA-N | |
Source | PubChem | |
URL | https://pubchem.ncbi.nlm.nih.gov | |
Description | Data deposited in or computed by PubChem | |
Canonical SMILES |
C1=CC(=C(C=C1OC2=C(C=C(C=C2I)CC(C(=O)OC3C(C(C(C(O3)C(=O)O)O)O)O)N)I)I)O | |
Source | PubChem | |
URL | https://pubchem.ncbi.nlm.nih.gov | |
Description | Data deposited in or computed by PubChem | |
Isomeric SMILES |
C1=CC(=C(C=C1OC2=C(C=C(C=C2I)C[C@@H](C(=O)O[C@H]3[C@@H]([C@H]([C@@H]([C@H](O3)C(=O)O)O)O)O)N)I)I)O | |
Source | PubChem | |
URL | https://pubchem.ncbi.nlm.nih.gov | |
Description | Data deposited in or computed by PubChem | |
Molecular Formula |
C21H20I3NO10 | |
Source | PubChem | |
URL | https://pubchem.ncbi.nlm.nih.gov | |
Description | Data deposited in or computed by PubChem | |
DSSTOX Substance ID |
DTXSID30858531 | |
Record name | 1-O-[O-(4-Hydroxy-3-iodophenyl)-3,5-diiodo-L-tyrosyl]-beta-D-glucopyranuronic acid | |
Source | EPA DSSTox | |
URL | https://comptox.epa.gov/dashboard/DTXSID30858531 | |
Description | DSSTox provides a high quality public chemistry resource for supporting improved predictive toxicology. | |
Molecular Weight |
827.1 g/mol | |
Source | PubChem | |
URL | https://pubchem.ncbi.nlm.nih.gov | |
Description | Data deposited in or computed by PubChem | |
CAS No. |
910907-23-2 | |
Record name | 1-O-[O-(4-Hydroxy-3-iodophenyl)-3,5-diiodo-L-tyrosyl]-beta-D-glucopyranuronic acid | |
Source | EPA DSSTox | |
URL | https://comptox.epa.gov/dashboard/DTXSID30858531 | |
Description | DSSTox provides a high quality public chemistry resource for supporting improved predictive toxicology. | |
Disclaimer and Information on In-Vitro Research Products
Please be aware that all articles and product information presented on BenchChem are intended solely for informational purposes. The products available for purchase on BenchChem are specifically designed for in-vitro studies, which are conducted outside of living organisms. In-vitro studies, derived from the Latin term "in glass," involve experiments performed in controlled laboratory settings using cells or tissues. It is important to note that these products are not categorized as medicines or drugs, and they have not received approval from the FDA for the prevention, treatment, or cure of any medical condition, ailment, or disease. We must emphasize that any form of bodily introduction of these products into humans or animals is strictly prohibited by law. It is essential to adhere to these guidelines to ensure compliance with legal and ethical standards in research and experimentation.